With any functioning brain cells at all in the stroke world this would be immediately seen as a way to objectively determine muscle disability and then determine objective results from any protocols used in rehab. But since there are NO functioning brain cells in stroke, nothing will occur.
Floating EMG sensors and stimulators wirelessly powered and operated by volume conduction for networked neuroprosthetics
Journal of NeuroEngineering and Rehabilitation volume 19, Article number: 57 (2022)
Abstract
Background
Implantable neuroprostheses consisting of a central electronic unit wired to electrodes benefit thousands of patients worldwide. However, they present limitations that restrict their use. Those limitations, which are more adverse in motor neuroprostheses, mostly arise from their bulkiness and the need to perform complex surgical implantation procedures. Alternatively, it has been proposed the development of distributed networks of intramuscular wireless microsensors and microstimulators that communicate with external systems for analyzing neuromuscular activity and performing stimulation or controlling external devices. This paradigm requires the development of miniaturized implants that can be wirelessly powered and operated by an external system. To accomplish this, we propose a wireless power transfer (WPT) and communications approach based on volume conduction of innocuous high frequency (HF) current bursts. The currents are applied through external textile electrodes and are collected by the wireless devices through two electrodes for powering and bidirectional digital communications. As these devices do not require bulky components for obtaining power, they may have a flexible threadlike conformation, facilitating deep implantation by injection.
Methods
We report the design and evaluation of advanced prototypes based on the above approach. The system consists of an external unit, floating semi-implantable devices for sensing and stimulation, and a bidirectional communications protocol. The devices are intended for their future use in acute human trials to demonstrate the distributed paradigm. The technology is assayed in vitro using an agar phantom, and in vivo in hindlimbs of anesthetized rabbits.
Results
The semi-implantable devices were able to power and bidirectionally communicate with the external unit. Using 13 commands modulated in innocuous 3 MHz HF current bursts, the external unit configured the sensing and stimulation parameters, and controlled their execution. Raw EMG was successfully acquired by the wireless devices at 1 ksps.
Conclusions
The demonstrated approach overcomes key limitations of existing neuroprostheses, paving the way to the development of distributed flexible threadlike sensors and stimulators. To the best of our knowledge, these devices are the first based on WPT by volume conduction that can work as EMG sensors and as electrical stimulators in a network of wireless devices.
Background
Clinically available neuroprostheses have been demonstrated to improve the quality of life of patients with neurological disorders or injuries, as these individuals gain significant functional improvement [1]. Additionally, some neuroprosthetic technologies have granted the possibility to perform intuitive control of robotic prostheses as they create an interface between the machine and the patient’s nervous system [2].
In the framework of the EXTEND collaborative project, funded by the European Commission and in which the authors participate, it has been defined the concept of Bidirectional Hyper-Connected Neural Systems (BHNS). The BHNS concept refers to systems consisting of minimally invasive communication links between multiple nerves or muscles in the body and external devices which may be interconnected between them (Fig. 1). The concept requires the deployment of dense networks of wireless active implantable medical devices (AIMDs) that can perform distributed electrical stimulation and sensing. These wireless AIMDs must communicate in real time with external devices and tools that process and analyze the neuromuscular activity and control the stimulation and the action of machines. BHNS could, for example, be used for (1) tremor management in essential tremor and Parkinson’s disease by tremor prediction using electromyography (EMG) [3], and subsequent intramuscular stimulation [4]; (2) performing functional neuromuscular stimulation [5]; or (3) interfacing with assistive wearable robots for spinal cord injury (SCI) and interfacing with prostheses providing control and artificial sensory feedback [6].
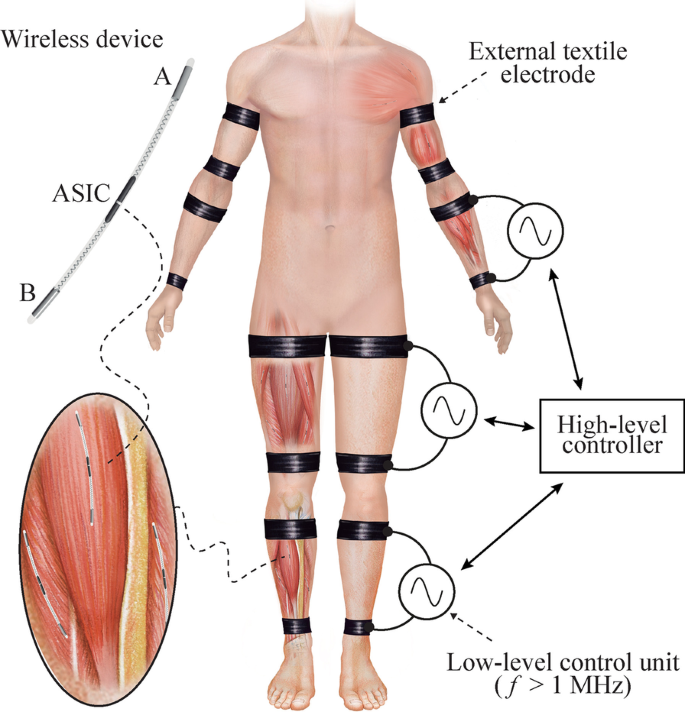
Schematic representation of a Bidirectional Hyper-Connected Neural System—BHNS. High frequency (> 1 MHz) volume conduction is used to wirelessly power and communicate with implantable devices for sensing and stimulation. The high-level controller of the external system communicates with several low-level control units that deliver current bursts through external textile electrodes. The envisioned wireless implantable devices will have a thread-like conformation to facilitate their deployment by injection. These devices will consist of a flexible body with two electrodes at their opposite ends (Electrodes ‘A’ and ‘B’) and their electronics will be integrated in an ASIC
Most neuroprostheses for chronic use, which are required to accomplish the BHNS concept, are implantable systems that consist of a central unit electrically connected to electrodes at target sites (e.g., cuff electrodes on peripheral nerves) by means of leads (i.e., wires) [7, 8]. However, these neuroprostheses present limitations that restrict their use. Those limitations, which are most detrimental in the case of motor neuroprostheses, often arise from their bulkiness and the need to perform complex surgical implantation procedures because of the leads [1, 9]. The leads also tend to fail due to mechanical stress, particularly in the case of motor neuroprostheses because electrodes are commonly deployed in long and mobile anatomical regions. As an alternative to these AIMDs based on central units, for the case of motor neuroprostheses, it was proposed and assayed the development of distributed networks of wireless intramuscular microstimulators that integrate the electronics and the electrodes [10, 11]. The devices communicate with external systems to perform neuromuscular stimulation aiming at motor restoration in patients suffering from motor paralysis [12]. The microstimulators were percutaneously implanted via injection, thereby avoiding complex surgeries. However, the devices were stiff and considerably large (diameters > 2 mm), making them unsuitable for their use in a dense network of wireless microstimulators.
The form factor of these wireless devices is mostly limited by the method used to power them. Batteries, with their intrinsic limited lifespan and large volume [13], are not suitable as primary sources of power in very small electronic implants. These floating implants, in clinical use or preclinically demonstrated, are typically powered by means of wireless power transfer (WPT) methods such as inductive coupling—the most established WPT method—[14,15,16,17], ultrasonic acoustic coupling [18,19,20] and capacitive coupling [21,22,23]. These methods have obtained high miniaturization levels, at the expense of link efficiency, penetration depth, or functionality. Recent reviews on these methods can be found in [13, 24,25,26].
In the last years we have proposed and demonstrated very thin microstimulators whose operation is based on rectification of volume conducted innocuous high frequency (HF) current bursts to cause local low frequency currents capable of stimulation [27, 28]. A portion of the HF current picked up by the devices is not directly rectified to perform stimulation but used to power the electronics of the implant (e.g., for control and communications) and, in this sense, it can be understood that the implants employ WPT based on volume conduction. In fact, in a series of recent works, we have advocated for, and studied, the use of volume conducted HF current bursts applied through textile electrodes to power elongated implants in general, not only stimulators [29,30,31]. Remarkably, according to the approach we propose, the implants can be conceived as thin, flexible and elongated devices suitable for implantation by means of injection [27]. The approach uses the body as an electrical conductor of innocuous and imperceptible HF current bursts that are applied by an external system using external textile electrodes. The implants do not require bulky components within their body to be electrically fed, allowing the integration of the electronics in an application-specific integrated circuit (ASIC). They pick up a small portion of the energy available through their two electrodes located at the opposite ends of the implant. The energy is used to power and bidirectionally communicate with the external system (Fig. 1). The elongated and ultrathin form factor allows the deployment of multiple implants in a single body region, favoring their use in dense networks of wireless AIMDs, such as those required by BHNS.
In the general architecture of BHNS, the external system will consist of one top-level controller that communicates with the wireless AIMDs through several external low-level control units that act as bidirectional gateways (i.e., protocol translators) between the implants and the external controller (Fig. 1). The low-level units apply bursts of HF currents to power and communicate with the wireless devices.
In Becerra-Fajardo et al. [27] we reported the development and in vivo evaluation of semi-rigid, thin and addressable stimulators based on this WPT approach. The injectable devices were made only of off-the-shelf components, and had an overall diameter of 2 mm. Although successfully demonstrated in animals, due to their invasiveness, these devices are not adequate to conduct assays in humans. Therefore, to be able to acutely demonstrate in humans the feasibility of the BHNS concept, within the framework of the EXTEND project it was decided to develop semi-implantable devices that consist of ultrathin and short intramuscular electrodes, made with thin-film technology, that are connected to a miniature electronic circuit to be fixed on the skin. Not only the electronics of the devices were upgraded for EMG sensing [32] but they were also upgraded for communication capabilities. Here we report the development and evaluation of these semi-implantable devices and the external system that powers and controls them. To the best of our knowledge, the wireless devices presented here are the only devices based on WPT by volume conduction that can work both as EMG sensors and as electrical stimulators to form networks of wireless devices.
More at link.
No comments:
Post a Comment