You described a problem but did nothing to solve it. WHAT THE FUCK ARE YOU IN STROKE FOR ANYWAY?
Impairments in the mechanical effectiveness of reactive balance control strategies during walking in people post-stroke
- 1Department of Biomedical Engineering, University of Southern California, Los Angeles, CA, United States
- 2Department of Biological Science, University of Southern California, Los Angeles, CA, United States
- 3Division of Biokinesiology and Physical Therapy, University of Southern California, Los Angeles, CA, United States
- 4Neuroscience Graduate Program, University of Southern California, Los Angeles, CA, United States
People post-stroke have an increased risk of falls compared to neurotypical individuals, partly resulting from an inability to generate appropriate reactions to restore balance. However, few studies investigated the effect of paretic deficits on the mechanics of reactive control strategies following forward losses of balance during walking. Here, we characterized the biomechanical consequences of reactive control strategies following perturbations induced by the treadmill belt accelerations. Thirty-eight post-stroke participants and thirteen age-matched and speed-matched neurotypical participants walked on a dual-belt treadmill while receiving perturbations that induced a forward loss of balance. We computed whole-body angular momentum and angular impulse using segment kinematics and reaction forces to quantify the effect of impulse generation by both the leading and trailing limbs in response to perturbations in the sagittal plane. We found that perturbations to the paretic limb led to larger increases in forward angular momentum during the perturbation step than perturbations to the non-paretic limb or to neurotypical individuals. To recover from the forward loss of balance, neurotypical individuals coordinated reaction forces generated by both legs to decrease the forward angular impulse relative to the pre-perturbation step. They first decreased the forward pitch angular impulse during the perturbation step. Then, during the first recovery step, they increased the backward angular impulse by the leading limb and decreased the forward angular impulse by the trailing limb. In contrast to neurotypical participants, people post-stroke did not reduce the forward angular impulse generated by the stance limb during the perturbed step. They also did not increase leading limb angular impulse or decrease the forward trailing limb angular impulse using their paretic limb during the first recovery step. Lastly, post-stroke individuals who scored poorer on clinical assessments of balance and had greater motor impairment made less use of the paretic limb to reduce forward momentum. Overall, these results suggest that paretic deficits limit the ability to recover from forward loss of balance. Future perturbation-based balance training targeting reactive stepping response in stroke populations may benefit from improving the ability to modulate paretic ground reaction forces to better control whole-body dynamics.
Introduction
People post-stroke have an increased risk of falls relative to neurotypical individuals (1) and this may be due, in part, to impairments in their ability to generate appropriate reactive strategies following a loss of balance. These impairments result from a combination of delayed muscle activation to external perturbations (2, 3), abnormal muscle activation patterns (4), and weakness (5). In addition, trips or slips, which commonly occur in the direction of walking, are one of the most prevalent causes of falls among people post-stroke (6). Although prior studies have examined the dynamics of backward losses of balance during stance (7, 8) and walking post-stroke (9–11), few have investigated the mechanics and recovery strategies following forward losses of balance during walking.
When responding to forward losses of balance during walking, neurotypical individuals adopt a sequence of reactive control strategies across multiple steps to counteract the forward rotation of the body (12). For example, when people trip over an obstacle, their first opportunity to recover balance involves modulating the support limb's push-off force to reduce forward angular momentum (13). Next, people often increase the length of the recovery step to reduce forward momentum while walking (12, 14–17). As a result, the ground reaction forces of the leading recovery limb and the perturbed trailing limb combine to generate a backward moment about the center of mass (CoM) and help arrest the forward rotation of the body (15).
However, sensorimotor deficits in people post-stroke may prevent them from executing successful reactions to forward losses of balance while walking. If a perturbation occurs during paretic stance, the paretic leg may be too weak to adequately support the body or it may lack the dexterity to properly regulate the body's momentum (18–21). Therefore, people post-stroke may not have sufficient time to step further forward with the non-paretic limb and arrest forward momentum. Conversely, if a perturbation occurs during non-paretic stance, they may have difficulty initiating a successful stepping response with the paretic leg to help restore balance due to deficits in paretic propulsion (22–24) and hip flexion (25). However, it has yet to be determined how paretic deficits impact the biomechanical consequences of reactive response to forward losses of balance or whether these effects differ following perturbations to the paretic vs. non-paretic limbs.
Here, our objective was to determine how stroke influences the biomechanical consequences of reactive control strategies following sudden treadmill accelerations (Figure 1). To counteract the increase in forward angular momentum following a perturbation, participants could use a combination of recovery strategies during the perturbation and recovery steps. First, they could reduce the forward angular impulse during the single stance phase following the perturbation. Second, they could increase the backward angular impulse generated by the leading limb during the recovery step. Finally, they could also decrease the forward angular impulse generated by the trailing limb during the first recovery step. We hypothesized that treadmill accelerations would cause larger increases in forward angular momentum in people post-stroke compared to neurotypical control individuals regardless of the side of the perturbation as post-stroke deficits may prevent these individuals from generating adequate reactive control strategies. We also expected that perturbations of the paretic leg would lead to greater increases in forward angular momentum than perturbations of the non-paretic side due to deficits in the ability of the paretic leg to support body weight (Figure 1A). When considering the biomechanical consequences of the reactive responses, we hypothesized that neurotypical participants would have larger contributions to the reduction of forward angular momentum from both the perturbed limb and the recovery limb compared with those of people post-stroke (Figures 1B,C). Lastly, we hypothesized that post-stroke participants would generate smaller reductions in forward angular impulse by the perturbed limb and larger increases in backward angular impulse using the recovery limb during the first recovery step following paretic vs. non-paretic perturbations (Figures 1B,C).
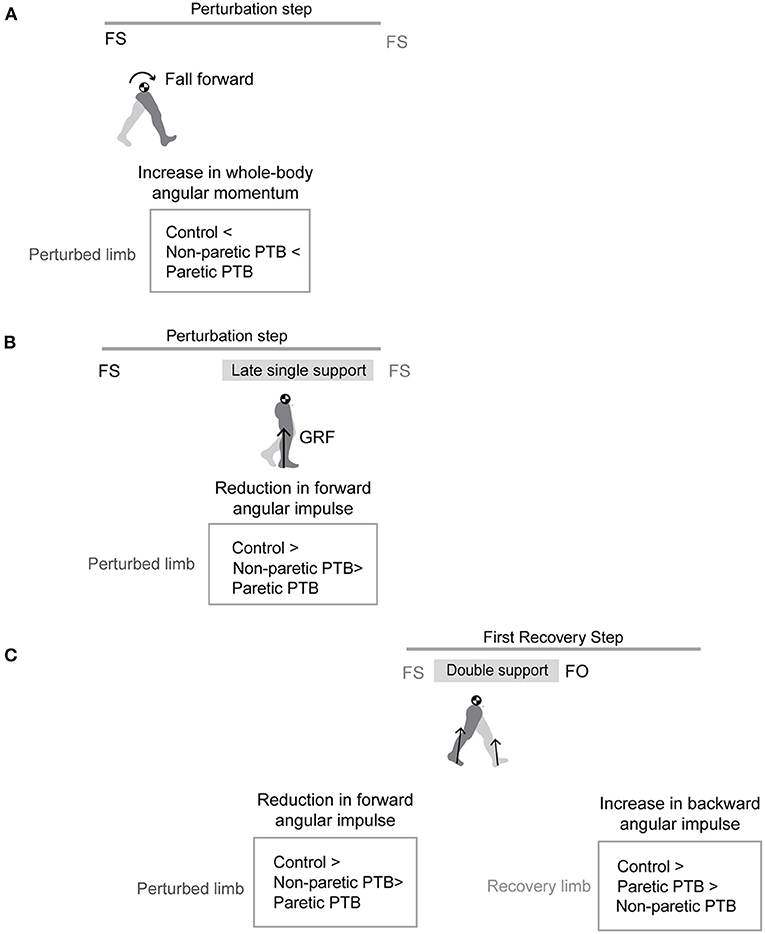
Figure 1. Graphical illustration of hypothesized differences in whole-body angular momentum and angular impulses. (A) Hypothesis for changes in whole-body angular momentum during the perturbation step relative to that measured during the pre-perturbation step. (B) Hypothesis for changes in angular impulse during the late single support phase of the perturbation step relative to that measured during the pre-perturbation step. (C) Hypothesis for changes in the trailing perturbed limb angular impulse and the leading limb angular impulse during the double support phase of the first recovery step relative to that measured during the pre-perturbation step (FS, foot strike; FO, foot-off; GRF, ground reaction force; PTB, perturbation).
No comments:
Post a Comment