Does your doctor have enough functioning brain cells to see this 226% improvement and immediately setup a protocol for its use for all stroke patients?
Do you prefer your doctor incompetence in this NOT KNOWING? OR NOT DOING?
Overnight olfactory enrichment using an odorant diffuser improves memory and modifies the uncinate fasciculus in older adults
- 1Department of Neurobiology and Behavior, University of California, Irvine, Irvine, CA, United States
- 2Center for the Neurobiology of Learning and Memory, University of California, Irvine, Irvine, CA, United States
- 3Department of Statistics, University of California, Irvine, Irvine, CA, United States
- 4Institute for Memory Impairments and Neurological Disorders, University of California, Irvine, Irvine, CA, United States
Objective: Cognitive loss in older adults is a growing issue in our society, and there is a need to develop inexpensive, simple, effective in-home treatments. This study was conducted to explore the use of olfactory enrichment at night to improve cognitive ability in healthy older adults.
Methods: Male and female older adults (N = 43), age 60–85, were enrolled in the study and randomly assigned to an Olfactory Enriched or Control group. Individuals in the enriched group were exposed to 7 different odorants a week, one per night, for 2 h, using an odorant diffuser. Individuals in the control group had the same experience with de minimis amounts of odorant. Neuropsychological assessments and fMRI scans were administered at the beginning of the study and after 6 months.
Results: A statistically significant 226% improvement was observed in the enriched group compared to the control group on the Rey Auditory Verbal Learning Test and improved functioning was observed in the left uncinate fasciculus, as assessed by mean diffusivity.
Conclusion: Minimal olfactory enrichment administered at night produces improvements in both cognitive and neural functioning. Thus, olfactory enrichment may provide an effective and low-effort pathway to improved brain health.
1. Introduction
There is a clear need for a new approach to the treatment of cognitive loss in older adults that takes little effort but is highly effective and affordable (Swedish Council on Health Technology Assessments, 2008). Environmental enrichment has long been studied in rats and mice, which can be enriched by placing them in a large cage with conspecifics, a running wheel, and regularly changing physical elements, rather than their restrictive box cage (Kempermann, 2019). The enrichment stimulates neuroplasticity that improves their human-like neurological symptoms in more than two dozen animal models of human neurological disorders (Nithianantharajah and Hannan, 2009; Hannan, 2014; Kempermann, 2019). Environmental enrichment also has more specifically been shown to ameliorate the human-like cognitive decline in animal models of aging (Valero et al., 2011; Patel, 2012). In lab animals, enhanced visual (Iaccarino et al., 2016), auditory (Martorell et al., 2019; Jung et al., 2023), and mastication (de Siqueira Mendes et al., 2021) stimulation, facilitates memory as it does in human older adults (Leon and Woo, 2018; Chan et al., 2022).
1.1. Olfactory enrichment alone improves brain and behavior in laboratory animals
Olfactory enrichment involves the daily exposure of individuals to multiple odorants and Veyrac et al. (2009) showed that olfactory enrichment alone could improve both memory and neurogenesis in the mouse brain. They further showed that novelty was the critical element in this kind of stimulation, as exposure to odorant mixtures did not produce these changes, while exposure to multiple odorants individually did. Rusznák et al. (2018) also showed that exposure to various essential oils alone for 30 min/day over 3 months induced neurogenesis in both the olfactory bulb and the hippocampus.
The olfactory system is the only sensory system that has direct projections to the limbic system which is crucial for memory and emotion, and which is the most relevant for this investigation (Haberly and Price, 1977), while the other sensory systems have indirect connections to this region via the thalamus. This unique access to the brain’s learning and memory systems may allow the olfactory system to prevent or reverse the deterioration of these systems via direct neural activation.
1.2. Loss of olfactory ability and the deterioration of cognition
As people age, the deterioration of their olfactory ability occurs before the deterioration of their cognitive abilities (Doty et al., 1984; Schaie et al., 2004). Additionally, olfactory loss results in a significant loss of both gray matter and white matter in human brains (Bitter et al., 2010a,b; Segura et al., 2013; Kollndorfer et al., 2014; Yao et al., 2014). COVID-19 typically results in olfactory loss and can result in long-term cognitive loss (Meng et al., 2020; Graham et al., 2021). Moreover, comparisons of MRI scans from individuals both pre-infection and post-infection have revealed neural deterioration that resembles a decade of aging in brain regions that receive olfactory-system projections (Douaud et al., 2022). Even chronic sinusitis has been shown to be associated with a decrease in gray matter in brain regions associated with learning and memory (Han et al., 2017).
1.3. Olfactory loss precedes or accompanies cognitive decline in dementia
Olfactory loss predicts the loss of gray matter in the hippocampus of older adults and continuing loss of olfaction predicts the further loss of hippocampal gray matter as they first develop Mild Cognitive Impairment (MCI) and then Alzheimer’s disease (Franks et al., 2015; Chen et al., 2021). Degradation of olfactory ability predicts which individuals with MCI will develop Alzheimer’s disease (Conti et al., 2013). In addition, olfactory dysfunction predicts cognitive dysfunction in humans (Choi et al., 2018) and the loss of olfactory function precedes or parallels the onset of a wide variety of other conditions such as: Parkinson’s disease (Ponsen et al., 2004; Meusel et al., 2010), Lewy body dementia (Ross et al., 2006), frontotemporal dementia, semantic dementia, frontotemporal dementia, corticobasal degeneration (Luzzi et al., 2007), Creutzfeldt-Jakob disease (Tabaton et al., 2004), alcoholism (Rupp et al., 2003), and schizophrenia (Kopala and Clark, 1990; Nguyen et al., 2010). Douaud et al. (2022) found that the same areas that deteriorate in older adults or adults with olfactory loss was seen in people who had experienced a COVID infection, even an infection with mild symptoms.
1.4. Olfactory stimulation restores olfactory function
Olfactory enrichment improves olfactory ability in humans with olfactory loss due to post-infection olfactory dysfunction (Konstantinidis et al., 2013, 2016; Damm et al., 2014; Geißler et al., 2014), head trauma (Huang et al., 2021), Parkinson’s (Haehner et al., 2013), or aging (Zambom-Ferraresi et al., 2021). These results were achieved with daily exposure to four odorants that represented the resinous, flowery, fruity, and aromatic odor groups. There are further improvements in olfactory ability with increased duration of exposure (Altundag et al., 2015; Konstantinidis et al., 2016), increased concentration of the odorants (Damm et al., 2014), and an increased number of odorants (Mahmut et al., 2020).
1.5. Olfactory enrichment changes human brain anatomy
Al Aïn et al. (2019) found that olfactory enrichment improved odor identification compared to that of visually enriched controls. Moreover, MRI analysis showed that olfactory enrichment led to increased cortical thickness in the right inferior frontal gyrus, the bilateral fusiform gyrus and the entorhinal cortex when compared to controls. Gellrich et al. (2017) found that olfactory enrichment given to people with olfactory deficiencies increased gray matter volume in the hippocampus and the thalamus, but no the brain regions. Similarly, Han et al. (2021) gave older adults olfactory enrichment for 7 months using 4 odorants twice/day, and patients had improved odor identification skills and larger cortical gray matter volume relative to controls. Sommelier students are exposed to dozens of novel odorants each day of their training. Using a longitudinal design, the brains of sommelier students were imaged with MRI at the start and end of their 18-month training and were compared with control students (Filiz et al., 2022). Olfactory enrichment of sommelier students increased olfactory bulb volume and it also increased the thickness of the entorhinal cortex. There were no significant changes in control group brains.
1.6. Olfactory enrichment improves cognition in humans
Haehner et al. (2013) showed that patients with Parkinson’s disease improved their verbal fluency after olfactory enrichment. Birte-Antina et al. (2018) provided olfactory enrichment for adults with 4 essential-oil odorants twice a day for 5 months. Controls solved daily Sudoku puzzles during that time. The olfactory-enriched group had a significant improvement of olfactory function, improved verbal function, and decreased depression symptoms. Oleszkiewicz et al. (2021) exposed 68 older adults either to 9 odorants twice a day or to no new olfactory stimulation for 3–6 months, and found the enriched olfactory experience produced improvements in cognitive abilities, dementia status, and olfactory function, relative to the control condition. Specifically, the Montreal Cognitive Assessment revealed a significant difference between the olfactory-enriched group and controls. They also found that the AD8 Dementia Screening Interview showed that olfactory-enriched participants had no increase in dementia symptoms over the course of the trial, while control participants had such an increase. Finally, an improvement on olfactory sensitivity was seen in olfactory-enriched individuals, but not controls. At the same time, Chen et al. (2022) did not find memory improvement in older adults with mild cognitive impairment after brief exposures to multiple odorants twice each day for 4 months. They did find that olfactory-enriched individuals increased frontal lobe activation but had no change in gray matter volume. In a similar study, Haehner et al. (2022) found that improvements in olfactory discrimination, increased thickness of the hippocampus, and improved global cognition were associated with increased thickness of the hippocampus, entorhinal cortex, and medial temporal lobes. Moreover, the change in the thickness of entorhinal cortex was positively associated with improvement of executive function.
1.7. Increased complexity of olfactory enrichment remarkably improves dementia
Cha et al. (2022) exposed older adults with moderate dementia either to 40 odorants twice a day for 15 days or to no olfactory enrichment. The olfactory-enriched group showed highly significant improvements in memory, olfactory identification, depression symptoms, attention, verbal fluidity, and language skills relative to controls.
1.8. Olfactory enrichment at night
While sniffing 40 odorants twice a day benefits patients with dementia, it is unlikely that they would be able to load, open, and close 80 sniff bottles each day. This problem would be expected even in older adults without dementia. Since it is important to get high levels of compliance for olfactory enrichment to obtain maximal benefits, we tested the idea that we could get enhanced neural and cognitive outcomes after minimal-effort olfactory enrichment at night.
The goal of the study was to determine whether participants retain or improve their cognitive ability after olfactory enrichment at night. We tested our hypothesis that the cognitive benefits of olfactory exposure on cognition may be found in its privileged access to brain areas and pathways relevant to olfaction and memory where it may be normalizing specific memory circuits. Specifically, we used diffusion weighted imaging to assess whether major limbic pathways (i.e., the uncinate fasciculus and the cingulum) are modified by olfactory enrichment. We focused on the uncinate in particular as a major pathway connecting the basolateral amygdala and the entorhinal cortex to the prefrontal cortex (Ebeling and Von Cramon, 1992; Thiebaut de Schotten et al., 2012; Von der Heide et al., 2013) and which plays a crucial role in learning and memory (Alm et al., 2016) and which deteriorates with age and Alzheimer’s disease (Morikawa et al., 2010; Fan et al., 2019). Importantly, a recent study demonstrated that the uncinate fasciculus is modified by a dance intervention as a form of environmental enrichment (Rektorova et al., 2020). This motivated our choice of the uncinate fasciculus as a target region of interest to test for the effect of olfactory enrichment.
2. Materials and methods
2.1. Participants
Participants were recruited from a list of interested older adults via the UCI Institute for Memory Impairments and Neurological Disorders’ Consent-to-Contact Registry. The participants were all community-dwelling older adults with no diagnosis of cognitive impairment or dementia. They received monetary compensation for their participation. Informed consent was given by all participants, all procedures were approved by the UC Irvine Institutional Review Board, and we conformed to the principles of the Helsinki Declaration. All participants were screened against major medical or psychiatric morbidities (including head trauma), substance abuse history, and any MRI contraindications, such as metal in the body. Inclusion and exclusion criteria are shown in Table 1. This trial was registered at ClinicalTrials.gov (Identifier: NCT03914989). Participants were 43 male and female, age 60–85, of good general health, with normal cognition, was defined as greater than or equal to 24 on the MMSE (see Figure 1 for subject participation flowchart). Participant characteristics are shown in Table 2.
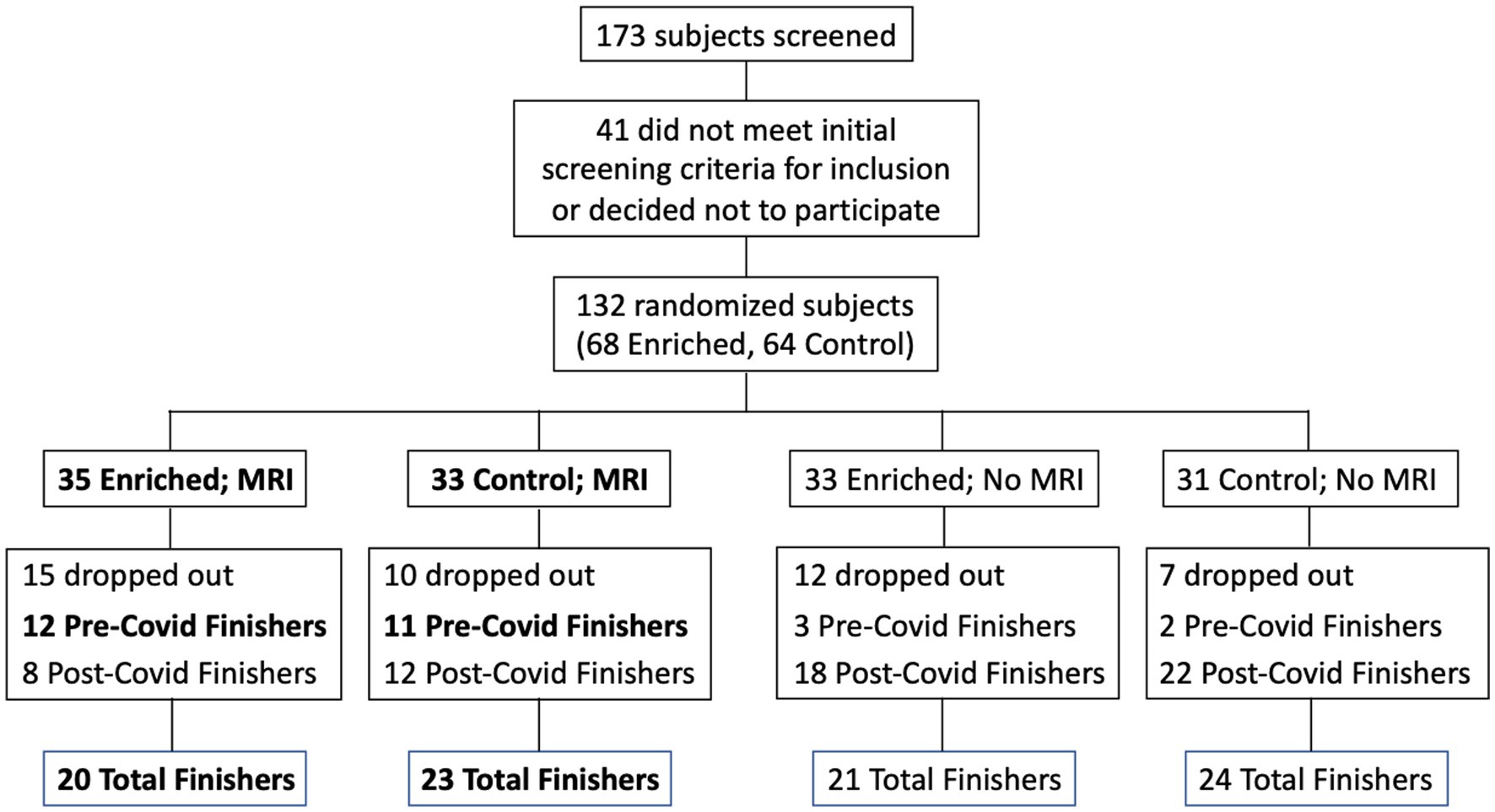
Figure 1. Flow chart for subject participation. Bold font denotes subgroups that were included in the statistical analyses (Pre-Covid Finishers, for neuropsychological assessments; and MRI Finishers, for MRI analyses).
We randomly assigned participants to receive daily exposure to essential oil scents (N = 20) or to a sham control condition with trace amounts of odorant (N = 23). CONSORT guidelines were adhered to in the analysis and reporting of study results. They were assessed for cognitive and olfactory abilities, as well as mental health status, at study entry and after 6 months. All participants received an odor diffuser to use at home for the 6-month duration of the intervention. The enriched group was exposed for 2 h every night over the course of 6 months, using a single odorant each night, and rotating through seven pleasant scents.
Participants provided Informed Consent, and completed a Background Information Questionnaire, a Fragrance Usage Survey, and a Daily Activities Questionnaire. The Background Information Questionnaire included age, education level, daily activities, medication, and emergency contact information. The Fragrance Usage Survey provided information regarding their typical daily odorant usage. The Daily Activities Questionnaire included the activities in which they participated that week. We used the Mini-Mental State Examination (MMSE) to confirm normal cognitive functioning.
2.2. Assessments and evaluations
We conducted a short neuropsychological test battery at baseline and after 6-month follow-up in all participants. To assess verbal learning and memory we used the Rey Auditory Verbal Learning Test (Rey, 1941), which assesses learning over five trials, followed by immediate and delayed recall tests. This test is also sensitive to both the integrity of the hippocampus and to the early detection of cognitive dysfunction (Saury and Emanuelson, 2017). Participants additionally completed three subsets of the Weschler Adult Intelligence Scale – Third edition (WAIS-III): the Digit Span Test (working memory) forward and backwards, and the Letter-Number Sequence test (planning and attention switching). We used Sniffin’ Sticks (Sensonics) to assess olfactory system function (olfactory identification, discrimination, and threshold) at baseline, allowing us to screen for olfactory abnormalities as well as to determine if olfactory enrichment enhanced olfactory performance.
2.3. Study intervention
Individuals assigned to the olfactory enrichment group were provided with an odorant diffuser (Diffuser World) and 7 essential oil odorants (rose, orange, eucalyptus, lemon, peppermint, rosemary, and lavender; from The Essential Oil Company, Portland, OR) in identical glass vials that each fit into the diffuser. They were asked to turn on the diffuser when they went to bed, and the odorant was released into the air during the night for 2 h when they first went to sleep. They rotated through the different odorants each night. Individuals in the control group also were provided with an odorant diffuser, and they followed the same regimen as the olfactory enrichment participants, however they were provided with bottles that contained distilled water with an undetectable, de minimis amount of odorant added. Participants were instructed to change the odorant bottle daily before they went to bed, and they continued this regimen at home for 6 months. Odorant bottles for both groups were labeled with the odorant name, and they were weighed prior to distribution, to obtain a baseline weight for the filled bottles, and then weighed again after 6 months to be sure that they were in use during the study. During each participant’s first visit, they smelled each of the scents used in the study and rated them on pleasantness and intensity.
We remained in contact with the participants during the first few days of the intervention to ensure adherence. We contacted each participant once a month during the intervention period to check on adherence, troubleshoot any issues, and inquire about any changes in health, or major life events. In addition, participants were asked to complete a daily Sensory Enrichment Log, which involved tracking the odorant exposures as they were completed, the time they went to sleep, and the approximate number of hours they slept that night.
2.4. Imaging methods
2.4.1. Acquisition
All MRI data were collected using a 3.0 Tesla Siemens Prisma scanner with a 32-channel head coil at Facility for Brain Imaging Research at UC Irvine. A high-resolution three-dimensional (3D) rapid-gradient echo (MP-RAGE) structural scan was acquired (0.8 mm3 isotropic, TR/TE = 2300/2.38 ms, 240 slices, FOV = 256 × 256, flip angle = 8o, slice orientation = sagittal, GRAPPA acceleration factor = 3). Diffusion data were acquired in two b-shells: 1500 s/mm2 and 3000 s/mm2, 64 non-collinear directions and a single volume with a b-value of 0 s/mm2. (TR/TE = 3500/102 ms, FoV = 218 mm, slices = 72, voxel size = 1.7 × 1.7 × 1.7 mm, Interleaved slices, Slice acceleration factor = 4).
2.4.2. Processing and analysis
DTI data collection and analysis were the same as for Granger et al. (2021). Motion correction and Eddy current correction were applied to raw data using FSL’s eddy tool (Andersson and Sotiropoulos, 2016). Corrected data were reconstructed using Q-spin Diffeomorphic Reconstruction (QSDR) function (Yeh and Tseng, 2011) in DSI Studio1, which uses a diffeomorphic algorithm to warp model-free orientation functions (ODFs) to Montreal Neurological Institute (MNI) space template. ODFs were reconstructed with the default diffusion sampling 1.25, which allowed modeling of crossing fibers at the intersection of the corticospinal tract and corpus callosum. Other reconstruction parameters included the following registration method: norm 7-9-7, eightfold ODF tessellation, number of fibers resolved. Output resolution was increased to 1 mm. Subject head motion was assessed by the eddy movement rms file exported from eddy (movement relative to the previous volume) and included as a nuisance regressor. T1-weighted MPRAGE scans were used to obtain intracranial brain volume using Freesurfer 6.0. The Johns Hopkins white matter atlas (in MNI space) in DSI studio contains various masks of regions of interest (ROIs) including limbic white matter regions, the uncinate fasciculus (UF) and the hippocampal cingulum bilaterally. We chose these two white matter pathways as major limbic-to-prefrontal tracts that are crucial to learning and memory.
Mean Diffusivity (MD), the average water diffusion rate within brain tissue, was extracted for each ROI. It was calculated as the mean of the three eigenvalues of the diffusion tensor vector (Salat, 2014). Generalized Fractional Anisotropy (GFA) values were extracted for each ROI and averaged across voxels. They were then averaged across both hemispheres. GFA is a model-free diffusion measure and is known to correlate with fractional anisotropy (FA) of the tensor model. GFA has been previously used to assess the structural integrity of complex tissues in a clinical setting, particularly when there are heterogeneous fiber tissues (Koh et al., 2018).
2.5. Impact of COVID-19 pandemic
Due to the COVID-19 pandemic, the UCI campus was closed in April 2020, and remained closed until the Fall of 2020. In addition, many participants did not feel comfortable entering the campus due to COVID-19 concerns even after the campus was officially open. As a result, participants who would have completed their 6-months of participation after April 2020 were either not able to return or chose not to return to campus for their second assessment. During the campus shutdown, contact was maintained with the participants who were impacted, and they were encouraged to continue their sensory enrichment, however, compliance was variable. When it became clear that the campus was going to remain closed for an extended period, we developed methods to remotely conduct the cognitive assessments using videoconferencing (Zoom app). When the campus re-opened and research participants were allowed back onto campus, participants who had received MRI scans at baseline received their second MRI scan.
The data set used for the cognitive assessment analysis was reduced due to a number of possible confounding issues including the different conditions present for the cognitive assessment testing at baseline (in office) and that given remotely (in their home using videoconferencing), the possible sensitivity of that testing to the immediate physical environment during the assessment, as well as the variable timing both between the date of the baseline assessment and the date of final assessment, and the date of their final assessment and the date they discontinued their sensory enrichment. Accordingly, in our data analysis for cognitive assessment, we only included individuals who had completed their 6-months of participation prior to the UCI shutdown (a total of 11 controls and 12 enriched). For the MRI analysis, we included everyone who returned to campus for their follow-up MRI despite the difference in time (range: 6–17 months; a total of 23 controls and 20 enriched).
2.6. Statistical analysis
The key analysis was a 2 × 2 mixed ANOVA with Time (Pre vs. Post) as the repeated (within subjects) measure and Group (Control vs. Treated) as the across-subjects measure. Given the matching of other variables including age, gender and years of education, we did not include these variables as covariates in the model. A two-sided P less than 0.05 was considered statistically significant. We used SAS9.4 for all statistical analyses.
3. Results
Although we used 7 odors in total with 2 h of olfactory stimulation each night, we only exposed participants to one odor each night, while others have used a minimum of four odors each day for olfactory enrichment (Pieniak et al., 2022). Despite the minimal variety of olfactory exposure each night, we observed a clear, statistically significant (Timepoint x Group interaction, F = 6.63, p = 0.02, Cohen’s d = 1.08, a large-size effect) 226% difference between enriched and control older adults in performance on the Rey Auditory Verbal Learning Test (RAVLT; last learning trial A5; Figure 2). This test evaluates verbal learning and memory, including proactive interference, retroactive interference, delayed recall, retention, and recognition memory. We found that 3 of 11 Controls improved, 1 of 11 stayed the same, 7 of 11 did worse. Among the Enriched group, 6 of 12 improved, 5 of 12 stayed the same, 1 of 12 did worse. Improvements with enrichment continued for retention trials (A6 and A7) following the interference list (B1), although those differences were just shy of statistical significance (F = 3.98, p = 0.06 for A6 and F = 3.69, p = 0.07 for A7). No other differences were observed in the other assessments (Table 3).
No comments:
Post a Comment