You can try to understand this or just turn it over to your doctor to explain. You need to know how close you are to a 'tipping point' after your stroke damage and how to prevent that.
Rat brains provide even more evidence our brains operate near tipping point
"Avalanches" in electrical activity between neurons provide a telltale signature.
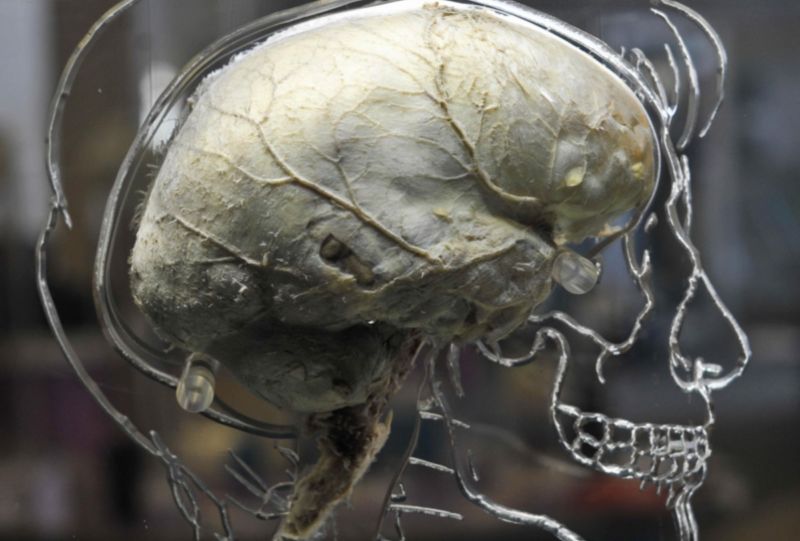
Enlarge /
A real human brain suspended in liquid within a human silhouette carved
into acrylic, on display at the Bristol Science Centre in England. New
research finds more evidence that the brain operates near a critical
point.
Ben Birchall/PA Images/Getty Images
The notion of so-called "self-organized criticality" dates back to a landmark paper in 1987, when the late Danish physicist Per Bak concluded that nature's exquisite order was the result of a kind of phase transition. That precise moment of transition is colloquially known as the "tipping point" or "critical point."
A brain's the thing
Typically, a classical phase transition only occurs when the temperature and pressure are just right for a given system. Self-organized criticality emerges spontaneously as the result of many local interactions between the many elements of a system, like millions of grains of sand running from the top to the bottom of an hourglass. The pile grows, grain by grain, until it becomes sufficiently unstable that the next grain to drop makes the pile collapse in an avalanche. The base of the pile widens, restoring stability, and the pile-up begins anew, until the sand pile hits the critical point again. Those avalanches follow a so-called "power law," meaning smaller ones happen more often than larger ones.Bak extended his ideas in a 1996 book, How Nature Works, applying the notion of self-organized criticality to earthquakes, financial markets, traffic jams, biological evolution, how galaxies are distributed in the universe, and the brain. In fact, he annoyed a group of neuroscientists in 1999 when he blithely informed them that he had figured out the fundamental principles behind how the brain works. Much like the pile of sand in an hourglass, he proposed that the brain teeters at that same critical point, a balance achieved by avalanches of electrical activity between neurons at all size scales. In other words, he proposed that electrical activity should follow a power law. The idea is that this critical state is a kind of "Goldilocks zone" that optimizes the transfer and processing of information in the brain while still maintaining stability.
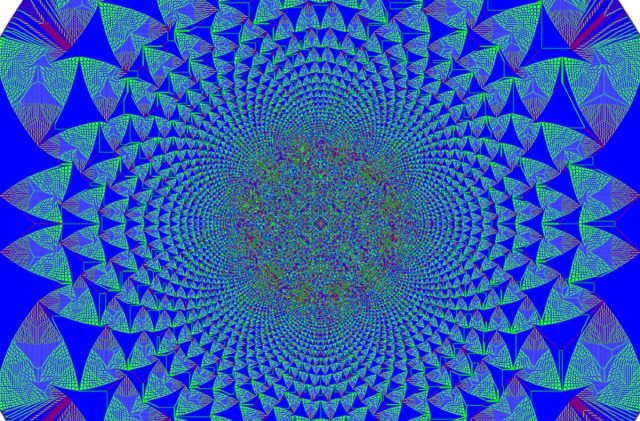
Enlarge /
The Bak–Tang–Wiesenfeld (Abelian) sandpile, here composed of 28 million
grains, is a famous model for self-organized criticality.
Signifying nothing?
All this was purely theoretical—and quite controversial—until 1992, when Swedish scientists conducted experiments with grains of rice pressed between glass plates, adding grains one by one and recording the eventual avalanches on camera. As Bak had predicted, there were more smaller avalanches than larger ones, following the telltale power law. In 2003, Indiana University biophysicist John Beggs and several colleagues were the first to demonstrate that slices of a brain's cortex showed evidence of an exponent relation. Add in evidence gleaned from other studies using magnetoencephalography, electroencephalography (EEG) recordings of the interactions between individual neurons, and computer simulations with fMRI imaging data of the brain's resting state, and it starts to look like proponents of the "brains at the tipping point" hypothesis might just have a case.The biggest issue is what one might call a "fine-tuning" problem: critical states in nature are generally fragile, in that a slight perturbation will move it away from the exact critical point. And the brain is an especially noisy system, so there would be many such perturbations. That's why Beggs and his Indiana University colleague Gerardo Ortiz have since argued that perhaps the brain hovers near, rather than precisely at, the critical point—think of it as more of a "quasi critical" phase space than a traditional tipping point. Similarly, Viola Priesemann of the Max Planck Institute for Dynamics and Self-Organization in Gottingen has argued that the brain operates in a slightly subcritical zone.
Not everyone is convinced. Among the most vocal critics of the brain operating in a critical state is Alain Destexhe, a theoretical neuroscientist at the National Center for Scientific Research (CNRS) in France. Among other concerns, power law distribution isn't sufficient evidence for criticality, since other processes in nature can mimic that signature. In a 2017 paper, he and co-author Jonathan Touboul proposed that the real sign of criticality would be the critical exponent relation.
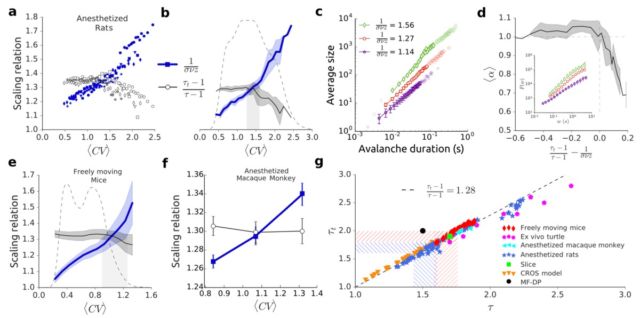
Enlarge / Signatures of criticality as a function of cortical spiking variability across several different species.
A.J. Fontenele et al./PRL
"This is mind-blowing"
Furthermore, if the brain operates near criticality, what kind of phase transition might be taking place? That's the question Antonio Fontenele and Mauro Copelli—both of the Universidade Federal de Pernambuco in Brazil—and various colleagues sought to answer with their experiments. They monitored the brains of lab rats as the rats moved from being asleep (thanks to a bit of anesthesia) and awake—technically, synchronized and unsynchronized states, respectively—and concluded that the rodent brains showed all the hallmarks of moving through a critical state as they cycled between sleep and wakefulness. Specifically, researchers saw spiking avalanches following a power distribution.In other words, "The transition is between unsynchronized and synchronized firing, this transition point is indeed critical, and the brain hovers around it," said Beggs, who was not involved with the experiments. "They had avalanches, too, and they satisfied the exponent relation. To me, this is mind-blowing."
Beggs was especially impressed with the second figure in this latest paper, which showed every data point following the exponent relation—stronger evidence for criticality than a power law distribution alone. That figure includes data from multiple experiments involving anesthetized rats, freely moving mice, anesthetized macaque monkeys, and even turtle brains. That's in keeping with a paper last year reporting on the recording neuronal activity in the brains of zebrafish, which showed similar evidence of hovering near criticality.
"This shows that a variety of quite different species, under different conditions, all line up near the critical point," said Beggs. "Usually with biological data you expect a cloud of points that may show some rough correlation. Here you get something noticeably different: there is order. This suggests that different brains are hovering around criticality, independent of many biological details." This should delight physicists, who love universality—that is, when an equation can capture the large-scale behavior of a complicated system like the brain—and irritate biologists. However, "It is very difficult to dismiss the idea of criticality in the cortex, given the data points lining up pretty nicely across species," said Beggs.
DOI: Physical Review Letters, 2019. 10.1103/PhysRevLett.122.208101 (About DOIs).
No comments:
Post a Comment