https://www.weforum.org/agenda/2016/08/this-is-what-a-single-neuron-looks-like-as-it-dies?
To learn how brain cells react to trauma, researchers are
delivering concussive impacts to cell cultures and imaging the aftermath
in real time.
The team used a custom-built laboratory device that can compress
neurons inside 3D cell cultures while using a powerful microscope to
continuously monitor changes in cell structure.
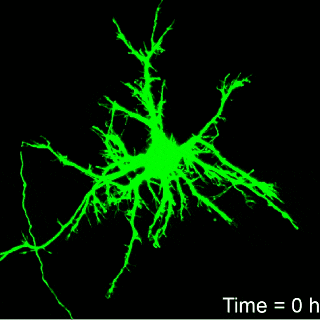
Image: Brown University
“This is the first study that applied this kind strain to brain
cells and followed them over time,” said Christian Franck, an assistant
professor in the Brown University School of Engineering and the paper’s
corresponding author. “We’re excited because we can finally get some
concrete information about when cells start to degenerate, when they
die, and what the process looks like.”
Among the study’s key findings was that after a compression event
with forces similar to those involved in traumatic brain injury (TBI),
it takes approximately six hours for neurons to develop irreparable
structural damage. That suggests that there may be a window for
therapeutic intervention aimed at minimizing further damage, Franck
says.
The study, published in Scientific Reports,
also found two different types of structural damage occurring in cells
following compression. One type, called diffuse axonal injury,
scientists know well as a hallmark manifestation of cellular TBI. But
the second form, which also led to cell death, has not previously been
associated with TBI. That finding suggests that the full extent of TBI
damage in the brain could be underestimated in some cases.
“We hope that this work provides some fundamental guideposts for
future research on TBI,” Franck says. “Once we understand how this works
at a basic cellular level, then we can start to think about adding the
complexity back in and understanding how it works in the brain.”
From 2D to 3D
What made the research possible was an experimental technique
Franck’s lab has been developing over the past few years. Most lab
studies on neuronal injury are done using two-dimensional cell cultures
grown on slides or petri dishes. To see what happens when cells are
placed under strain, researchers either stretch them or expose them to
shearing forces. But 2D techniques can’t evaluate compressive force,
which is known to be important in blows to the head.
“We wanted to add this other facet of compression to our laboratory work,” Franck says. “The only way you can do that is in 3D.”
Franck and his colleagues grew rat neurons in 3D collagen cultures
about the diameter of a quarter. They then placed the cultures in their
device, which has a hydraulic piston apparatus that sits atop a
laser-scanning microscope. The piston delivers precisely tuned
compressive force to the culture, while the microscope generates
continuous images of cell structures.
Hours until death
That real-time observation was a key part of the work, Franck says.
“If we can look at this continuously, then we can get a much better
idea of when permanent damage begins and when cells finally die.”
Researchers have known for a while that much of the damage and cell
death in TBI does not occur at the moment of impact. Instead, there
appears to be a biochemical cascade that, over a period of time, causes
damage to accrue in the cells and eventually leads to death. One
problem, however, is that nobody knows exactly how long that process
takes after a compressive strain, or whether it occurs slower or faster
depending on the severity of the impact.
Franck’s work found that it take about six hours for neurons to
show signs of irreparable damage from the amount of compression thought
to be typical of blows to the head. But the timing from the onset of
damage to cell death varied according to the amount of compression. For
example, cells exposed to a 7 percent strain died after about 12 hours,
while those exposed to a 12 percent strain died in around seven hours.
“Our system doesn’t have nearly the complexity of a real brain, so
we’re not saying that it plays out exactly this way in TBI patients,” he
says. “But we wanted to start simply and get fundamental data on how
neurons respond to these strains. We think it provides a good starting
point for further research.”
Different kinds of damage
A particular advantage to Franck’s device is that is that it
enables precise control over the total amount of compression and how
long it is applied (called strain rate). The study showed that both of
those factors influenced cellular injury in different ways. While the
total amount of compression was responsible for the timing of cell
death, strain rate was responsible for how many cells were damaged and
what kind of damage they suffered.
At the higher strain rates used in the study, neurons developed
diffuse axonal injury (DAI)—the hallmark of TBI in which cellular
appendages called axons swell and eventually break, forming bubbles or
“blebs” at the breaking points. But at slower strain rates, the
structural damage took a different form. Instead of breaking and forming
blebs, the axons and neurite extensions simply retracted as the cells
died.
“Pathologists look for blebs and other signs of DAI to assess
whether a TBI has occurred and how widespread the damage is,” Franck
says. “But what this suggests is that they may be missing this other
type of morphology.”
The effect of strain rate is important because while scientists
know generally how fast TBI-related impacts are (in the National
Football League, for example, impacts are about 15 milliseconds), it’s
not clear how that energy wave is transmitted through the brain. The
strain rate applied to the outside of the head could be very different
from what is transmitted through the brain, and this study suggests that
the manifestation of injury could be different depending on the rate.
Franck and his colleagues plan to continue using their apparatus to
explore cellular TBI. In the future they intend to use more complex
culture media in order to better simulate the differing tissue
structures in the brain. They are also interested in using their device
to evaluate possible therapeutic measures.
“The nice thing is we have a very well-controlled system to cause
injury that can also be used to evaluate therapeutic interventions,”
Franck says. “We can inject drugs into the culture, for example, and see
how the cells respond.”
Franck hopes that the results from these kinds of lab studies will
eventually guide clinical research aimed at reducing the devastating
effects of TBI.
No comments:
Post a Comment