You mean your mentors and senior researchers are so fucking incompetent they missed this from May 2016? My god, is there a need for a smidgen of stroke leadership.
TOOTH (The Open study Of dental pulp stem cell Therapy in Humans): Study protocol for evaluating safety and feasibility of autologous human adult dental pulp stem cell therapy in patients with chronic disability after stroke May 2016
Dental Pulp Stem Cells: An Attractive Alternative for Cell Therapy in Ischemic Stroke
- 1Department of Neurology, Dalian Municipal Central Hospital Affiliated to Dalian Medical University, Dalian, China
- 2Department of Pharmacy, Dalian Municipal Central Hospital Affiliated to Dalian Medical University, Dalian, China
- 3School of Life Sciences, University of Warwick, Coventry, United Kingdom
Ischemic stroke is a major cause of disability and
mortality worldwide, but effective restorative treatments are very
limited at present. Regenerative medicine research revealed that stem
cells are promising therapeutic options. Dental pulp stem cells (DPSCs)
are autologously applicable cells that origin from the neural crest and
exhibit neuro-ectodermal features next to multilineage differentiation
potentials. DPSCs are of increasing interest since they are relatively
easy to obtain, exhibit a strong proliferation ability, and can be
cryopreserved for a long time without losing their multi-directional
differentiation capacity. Besides, use of DPSCs can avoid fundamental
problems such as immune rejection, ethical controversy, and
teratogenicity. Therefore, DPSCs provide a tempting prospect for stroke
treatment.
The past decade has witnessed intense advancement and
tremendous therapeutic achievements in the ability to diagnose and treat
stroke, a cerebrovascular disease of which 87% is ischemic in nature.
Nevertheless, stroke remains a major cause of disability, morbidity, and
mortality worldwide, and constitutes a major socioeconomic problem (1, 2).
Ischemic stroke, due to partially or completely blocked blood flow in a
cerebral artery, causes ischemic necrosis of brain tissue seriously
impairing the health of affected individuals. The main therapeutic
strategy for ischemic stroke is timely recanalization. This can either
be achieved by tissue-type plasminogen activator application or
mechanical thrombectomy. Particularly the latter can be applied up to 24
h after stroke in patients exhibiting a penumbra, and has
revolutionized acute stroke treatment. However, the absolute number of
patients qualifying for recanalization remains very low (3, 4). Hence, additional treatment approaches being effective beyond the first hours after stroke onset are urgently required.
Stem cell transplantation is a promising strategy to restore neurological function after stroke (5).
Experimental stem cell transplantation in animals showed that numerous
cell populations can improve functional recovery by a broad spectrum of
mechanisms (6–8).
Several kinds of stem cells are currently considered for therapy. These
include embryonic stem cells (ESCs), fetal stem/progenitor cells,
induced pluripotent stem cells (iPSCs), and adult stem cells. While
embryonic or induced pluripotent stem cells exhibit a tremendous
differentiation potential, they may also inherit a risk for tumor
formation (9).
The use of embryonic stem cells or fetal stem/progenitor cells raises
ethical concerns. Adult stem cells show a limited proliferation and
differentiation potential, but can still be beneficial after stroke due
numerous mechanisms beyond tissue restoration. They are further believed
to be safer in clinical application and their use is ethically less
challenging (9–13).
Recent systematic reviews and meta-analyses on the most
prominent adult stem cell therapy candidates, mesenchymal stem cells
(MSCs), presented evidence that MSCs improve the outcome after stroke in
animals (14) and patients, and confirmed the safety and feasibility of the approach (15).
Nevertheless, there is still a lack of adult (stem) cells that can be
derived from an autologous source, and may exhibit therapeutic abilities
beyond those of MSCs.
Dental Pulp Stem Cells (DPSCs): A New Source of Adult Stem Cells
The dental pulp is a soft tissue located in the center of
teeth. It comprises blood vessels, neural fibers, and connective
tissue. The dental pulp contains both mesenchymal and ectodermal tissue
as well as neural crest cells (16).
Limited dentinal repair in the postnatal organism relies on specialized
precursor cell populations residing in the dental pulp tissue. Gronthos
et al. first reported the isolation and characterization of stem cells
from dental pulp tissue of the third molar in 2000 (17). DPSCs are ectoderm-derived stem cells, originating from migrating neural crest cells (Figure 1).
They are a subpopulation among dental pulp cells (DPCs) which possess
MSC properties, such as a fibroblast-like morphology, adherence to a
plastic surface, as well as surface marker expression, proliferation and
colony forming behavior similar to that of MSCs (18, 19).
It is not clear whether or not DPSCs are a kind of MSC population.
Given their differentiation abilities as reviewed below, it might be
assumed that DPSCs are a more naïve stem cell population that also, but
not exclusively, exhibits MSC properties. A major benefit of DPSCs is
that they can be isolated during routine dental procedures such as the
eruption of deciduous teeth or extraction of impacted wisdom teeth (20)
in simple and autologous fashion without ethical concerns. Another
primary advantage of DPSCs is their potential for cell banking. Several
studies have demonstrated that DPSCs retain their stem cell properties
after long cryopreservation (21, 22).
This is essential as cryopreservation can impact therapeutic capacities
of other adult stem cell-containing populations in stroke (23).
In addition, DPSC cultures can be established from extracted human
molars with high efficiency, even after the whole tooth has been
cryopreserved for up to 1 month (24).
DPSCs also exhibit a multilineage differentiation potential into
chondrocytes, adipocytes, odontoblasts, and potentially even neural-like
cells (25–28).
FIGURE 1
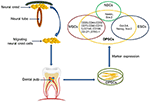
Currently, there are no specific
markers that uniquely define DPSCs. In general, DPSCs, as a
heterogeneous population, express a variety of markers similar to MSCs (Table 1) (Figure 1), and do not express hematopoietic markers such as CD14, CD19, CD34, and CD45 (18, 26, 29–32). DPSCs isolated by their high proliferative potential tend to include a large population of cells expressing CD44+, CD90+, and CD166+.
However, DPSCs also express stemness-related markers similar to ESCs
such as Oct-3/4, Nanog, and Sox-2, as well as the cytoskeleton-related
markers nestin and vimentin (Figure 1) (33–35).
They further express insulin-like growth factor 1 receptor (IGF1R)
which is regarded as a pluripotency marker in ESCs. DPSC-secreted IGF1
interacts with IGF1R through an autocrine signaling pathway to maintain
self-renewal and proliferation potential (36).
TABLE 1
In addition, DPSCs (as neural
crest-derived stem cells) not only express a number of neural stem cell
(NSC) associated markers including nestin (26, 37) and Sox2 (38) (Figure 1),
but also express low basal levels of markers associated with mature
central nervous system cell types, including the neuronal markers
βIII-tubulin, microtubule-associated protein 2 (MAP2), neurofilaments
(NF) (33, 39), NeuN (40), the astrocytic marker glial fibrillary acidic protein (GFAP) (26, 33), and oligodendrocyte-associated CNPase (33).
Taken together, this suggests that DPSCs can indeed differentiate into
neuron-like cells under appropriate conditions, and differentiated cells
even exhibit typical electrophysiological properties after neuronal
differentiation (41, 42).
DPSCs as a Potential Candidate for Therapy of Neurological Diseases
Brain-derived NSCs are considered a promising population
for stroke treatment due to their ability to self-renew and to
differentiate into neural cells types (neurons, astrocytes,
oligodendrocytes) (43). However, autologous harvest of adult human NSCs requires neurosurgical procedures due to their brain parenchymal residence (44),
while allogeneic or even xenogenic NSCs grafting imposes the risk of
graft rejection and additional immunological damage. Only a limited
number of clinical trials currently explore the potential of NSCs for
stroke treatment because of these limitations.
Adult stem cells or stem cell-containing populations are
more frequently applied in translational research. As stated above,
DPSCs share many biological characteristics with MSCs including bone
marrow MSCs (BM-MSCs), adipose tissue-derived stem cells (ADSCs) and
umbilical cord MSCs (UC-MSCs) but there are some variations in their
proliferation potential (17, 27, 45), differentiation potential (17, 27, 46), immunomodulatory activity (27), secretome characteristics, and secretory capacity (47–49). Specifically, DPSCs have a higher proliferation rate and a greater clonogenic potential than MSCs (17, 45). Next to DPSCs, the DPC population also contains a higher number of stem/progenitor cells as compared to bone marrow (50).
This may be attributed to the developmental state of the respective
tissues. All teeth, even the permanent molars, are generated early in
individual development and rest in the jar until they erupt. Abilities
and capacities of stem cells may be much better preserved in tissue with
a slow turnover such as the dental pulp when compared to BM, which
exhibits a tremendous turn-over throughout life apart from some niches.
DPSCs maintain their high rate of proliferation even after extensive
subculturing.
Like MSCs, DPSCs can differentiate into cells of mesenchymal and non-mesenchymal tissues in vitro and in vivo.
However, DPSCs exhibit stronger odontogenesis and neurogenesis
capabilities, in turn being not as potent to produce adipogeneic,
osteogeneic and chondrogeneic tissue than BM-MSCs (51) and ADSCs (46).
Besides, DPSCs also have immunomodulatory capacities exceeding those of
BM-MSCs, for example a higher suppression rate of T lymphocyte growth (17, 27).
DPSCs exhibit superior neuroprotective and
neuro-supportive properties in neurological injuries and pathologies as
compared with BM-MSCs and ADSCs (52).
This might be related to a higher expression of trophic factors
including brain derived neurotrophic factor (BDNF), glial cell-derived
neurotrophic factor (GDNF), nerve growth factor (NGF), vascular
endothelial growth factor (VEGF), and platelet derived growth factor
(PDGF) in DPSCs as compared to BM-MSCs (47, 48), although the spectrum of growth and trophic factors secretion is similar (53). DPSCs also express higher quantities of CXCL14 and monocyte chemoattractant protein 1 (MCP-1) than ADSCs (49).
Besides, the DPSC secretome contains higher concentrations of RANTES,
FRACTALKINE, fms-related tyrosine kinase 3 (FLT-3),
granulocyte-macrophage colony-stimulating factor (GM-CSF), and MCP-1
than the BM-MSCs secretome (54).
DPSCs show higher angiogenic and neurogenic potentials in ectopic
transplantation models compared to BM-MSCs and ADSCs, and exhibit the
highest migration capacity. Transplantation of DPSCs in a mouse hindlimb
ischemia model produced higher blood flow and capillary density than
transplantation of BM-MSCs and ADSCs, which being associated with
superior recovery of limb movement abilities and reduction of ischemic
hindlimb damage (55).
DPSCs also mediate stronger anti-apoptotic effects in a
microenvironment challenged by oxidative and serum deprivation than
BM-MSCs, ADSCs and UC-MSCs (45).
Cell size and diameter are important for safety after
intravascular delivery as they are the major, but not the only,
determinants of vascular obstruction and complications (56) (Table 2). Previously reported studies showed that the cell diameter of human DPSCs is around 15–16 μm (59), which is comparable to NSCs (57, 58) but slightly smaller than for most MSC populations (57, 60) including human BM-MSCs (61).
Still this means that one has to expect a considerable pulmonary
passage filtering effect after intravenous delivery, as well as a risk
for microembolism after intraarterial administration (62).
Hence, thorough investigations identifying the optimal route of DPSC
administration by considering safety and efficacy aspects are
recommended in DPSC translational research.
No comments:
Post a Comment