So you're telling us what we already know and produced nothing new for survivor recovery. Useless.
Microbiome-Based Interventions: A New Prospect in Post-Stroke Rehabilitation
Submitted: February 14th, 2022Reviewed: February 28th, 2022Published: April 16th, 2022
DOI: 10.5772/intechopen.103976
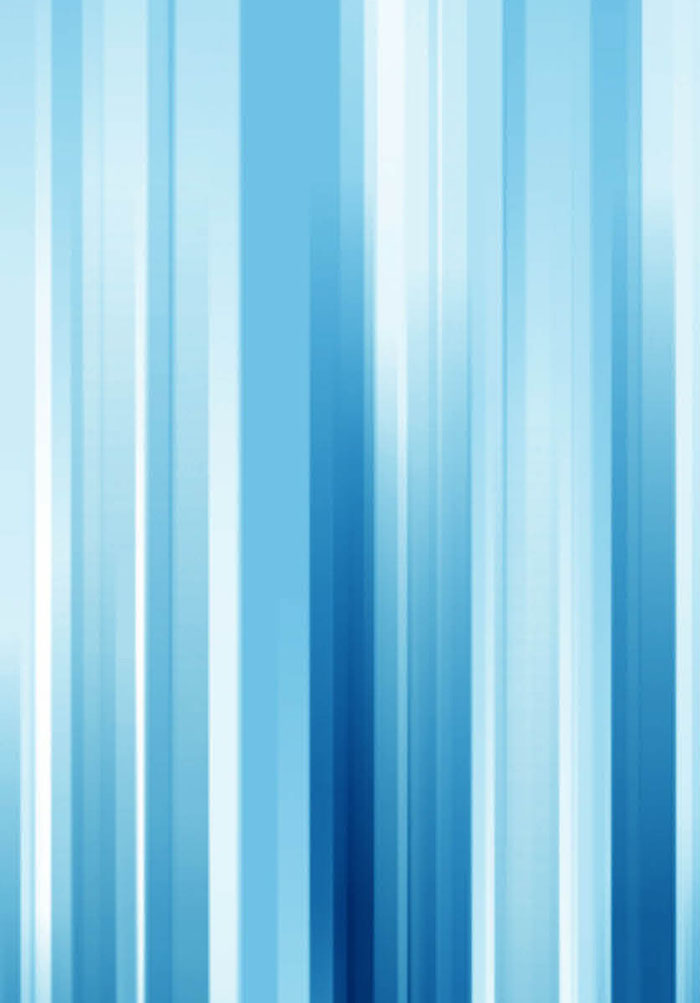
From the Edited Volume
Post-Stroke Rehabilitation [Working Title]
Dr. Pratap Sanchetee
Chapter metrics overview
6 Chapter Downloads
Abstract
Post-stroke rehabilitation remains the preferred therapeutic option for stroke survivors due to its unrestrictive therapeutic window of unlimited lifelong applicability. However, post-stroke rehabilitative interventions are still far from ideal and optimal recovery from lost functions after stroke. This heralds the search for strategies to complement rehabilitative interventions. Expanding the armamentarium of the existing post-stroke rehabilitation strategies will go a long way towards the attainment of optimal functions lost due to stroke. One of the promising emerging trends in stroke is cherished within the microbiome present in the gastrointestinal system. There is bidirectional communication between stroke and gut microbiome via gut-brain axis, and plethora of evidence pointed that modulation of this axis impact on stroke outcome, as well as evidence linking gut microbiome in modulation of brain neuroplasticity. Herein, we explored evidence that will support future research and perspectives into the potentiality of microbiome-based interventions as an integral part of post-stroke rehabilitation. Findings support the premise of the function of gut microbiome in brain neuroplasticity, and this could be fundamental towards translating similar phenomenon in human stroke to promote brain neuroplasticity in complement with post-stroke rehabilitation.
Keywords
- microbiome
- microbiota
- microbiota gut-brain axis
- stroke
- post-stroke rehabilitation
- neuroplasticity
Author Information
Show +1. Introduction
A stroke occurs due to interruptions in blood supply to the brain, and over the years stroke consistently constitutes the second most common cause of mortality after cardiovascular diseases and a foremost root of chronic adult disability [1, 2, 3]. Individuals that survive stroke experience a multifaceted range of impairments as a result of loss of brain function due to the infarct, with acute clinical treatment of those impairments tailored towards rescuing the larger component of potentially affected but yet viable neurons in the ischemic penumbra that surround the ischemic core [4]. However, the approved strategy to salvage neurons in the ischemic penumbra using recombinant tissue plasminogen activator (rtPA) and mechanical thrombectomy remains largely unsatisfactory and is only available to an only small fraction of about 5% of stroke patients due to narrow time of applicability within 4.5 h of stroke onset [5]. Moreover, despite advancement in understanding the mechanism of neuronal demise after stroke and the corresponding development of neuroprotective agents, translating findings from bench to bedside has been largely disappointing, as about 70 agents tested in more than 140 clinical trials have all been unsatisfying [6].
Therefore, post-stroke rehabilitation aims at restoring functional impairments as well as promoting better compensation of the resulting defects after stroke remains a favorite therapeutic option against stroke due to its relative advantage of unrestrictive time of applicability [7]. Rehabilitative interventions are principally based on modifying and boosting the neuronal plasticity processes [8, 9]. Neuroplasticity is a general term used to denote the life-long natural capability of the nervous system to act in response to intrinsic or extrinsic stimuli by rearranging itself in terms of structural, functional, and molecular form [10]. There are numerous processes of neuroplasticity that allow for neuronal reorganization, which includes neurogenesis, gliogenesis, angiogenesis, synaptogenesis, dendritic arborization, axonal sprouting, long-term potentiation (LTP), enrollment of other pathways, strengthening of functionally silent synapses. Thus, the categorization into neuronal plasticity (neurogenesis), synaptic plasticity (LTP), and global plasticity is often used to take into account the various organizational level of neuroplasticity. Brain injury, due to stroke, is one of the potent stimuli that lead to the activation of neuroplasticity processes to initiate spontaneous recovery. The rehabilitative interventions take advantage of this endogenous brain capacity to promote it as well as remodel it in the right direction to achieve maximum recovery from functional impairment after stroke [11, 12].
Nevertheless, it was estimated that about 50% of individuals that survived stroke are left with residual functional impairment even with rehabilitative interventions [13]. This prompted the search for strategies that enhance neuroplasticity to complement rehabilitative interventions. Various strategies to achieve that using transcranial direct current stimulation, functional electrical stimulation, deep brain stimulation, brain-derived neurotrophic factor (BDNF) therapy, Statins therapy, erythropoietin (EPO) therapy, phosphodiesterase type 5 inhibitors, and vascular endothelial growth factor (VEGF) therapy have all been under investigation [4]. But considerations for gut microbiome-based interventions to enhance neuroplasticity and supplement neuro-rehabilitation of stroke have not been explored. This is despite the fact that there exists numerous evidence indicating mechanistic link implicating gut microbiome in the pathogenesis of stroke and mediating through gut microbiome to impact on stroke outcome [14, 15, 16, 17], as well the role microbiome as a potential modulator of brain neuroplasticity [18].
Gut microbiome is composed of a complex community of trillions of microbes, consisting of bacteria, fungi, viruses, archaea, and protozoa, with bacteria constituting more than 90% of the gastrointestinal ecosystem [19, 20, 21, 22]. The mechanism through which the gut microbiome mediates an integral role in the pathogenesis of stroke has been well established [23]. Brain lesion due to stroke together with the effect from stroke-induced immunosuppression caused harmful consequences on gut microbiome via top-down signaling of microbiota gut-brain axis. Such consequences include dysbiosis, imbalance in resident intestinal immune function, and intestinal barrier dysfunction [24]. The bottom-up signaling of microbiota gut-brain axis through microbiome associated effect in up-regulation in pro-inflammatory immune cells and cytokines, as well as an increase in permeability of microbes and their components, lead to compounding effect on stroke injury by escalating neuro-inflammation and unsettling in integrity of blood–brain barrier (BBB) [25]. In this paper, therefore, we explored evidence that will support future research and perspectives into the potentiality of microbiome-based interventions as an integral part of post-stroke rehabilitation.
2. Post-stroke rehabilitation and neuroplasticity
Post-stroke rehabilitation entails healthcare that manages post-stroke disability and the underline condition that cause or accompany the stroke, with the goal that focuses on reducing the disability and enhancing the performance in activities of daily living (ADL). Because post-stroke rehabilitation objectives are complex and dynamic involving measures to avoid further decline in function, optimize the existing function, and reach for the highest possible level of independence (physically, psychologically, socially, and financially) possible, multidisciplinary interventions from a number of disciplines are employed [26]. Post-stroke rehabilitation guidelines are therefore developed based on evidence-based best data to improve and support the best clinical rehabilitation of stroke [27, 28]. Nevertheless, even with the best clinical practice in post-stroke rehabilitation, certain populations of stroke survivors are still living with residual disabilities. Rehabilitation as a process generally explores the basis for brain recovery from impairments, which are adaptation, restitution, and neuroplasticity [29].
Recovery in function in post-stroke brain depends majorly on neuroplasticity. Neuroplasticity entails the inherent capability of the CNS to change structurally and functionally as a result of new experiences [30]. There exist many techniques to detect neuroplasticity in the human brain. The most frequently used include functional magnetic resonance imaging (fMRI) which assesses changes in activation and recruitment of brain regions and noninvasive brain stimulation which can detect a change in volume, location, and excitability of motor cortical maps [31]. The basic processes in learning-related plasticity include the amplification of existing neuronal connections, as well as the formation of new connections to support learned behaviors [32]. These processes are then followed by synaptic pruning of the connections as skill and preferential pathways develop. For this, one of the current approaches to optimize the functional benefits of post-stroke motor rehabilitation is by focusing on interventions that encourage motor learning-related neuroplasticity [31].
The molecular level of synaptic plasticity has been extensively studied. In synaptic plasticity, there is exocytosis of neurotransmitters to modulate synaptic plasticity either at monosynaptic or multi-synaptic level, thus neurotransmitter-receptor binding is critical to synaptic plasticity [33]. Within the cortex, glutamate receptors play a key role, as glutamate is the most important excitatory neurotransmitter. The arrival of impulses from neighboring neurons leads to the activation of metabotropic glutamate receptors. This allows for calcium influx which consequently engages machinery for protein synthesis and permanently changes postsynaptic neurons [34].
3. Microbiota gut brain axis and stroke
Gut-brain axis has recently been increasingly recognized as one of hallmark biological processes linked with the pathophysiology of stroke [35]. Gut-brain axis primarily refers to the network of biological connections that link the gastrointestinal tract (GIT) and the central nervous system (CNS), allowing for bidirectional communication between the two [36, 37]. By implication, the bidirectional communication here indicates two-way communication implying that while on hand the brain modulates the regulation of gut activities (top-down signaling); on the other hand, the gut also can regulate the functions of the brain (bottom-up signaling). These regulatory signals between the two are executed through multiple mechanisms, including neural, endocrinal, immunological, and metabolic pathways. The components of the brain that drive gut-brain axis include the hypothalamus, medial prefrontal cortex, nucleus of the solitary tract in the medulla, and amygdala among others [38]. The gastrointestinal components include the intestinal cells and the microbes within the gut termed microbiota. The term gut microbiota refers to complex assembly of microbes residing within the human gastrointestinal tract [39]. The microbes within microbiota community are constituted by extraordinary densities of 100 trillions of microorganisms, including more than 1000 species, of mostly bacteria, but also fungi, archaea, protozoa, and viruses, together with their collective genomes termed microbiome [21, 22]. Because the gut microbiota contributes to the major components involved in gut-brain axis, the term microbiota gut-brain axis is used as an extension to recognize the integral position of gut microbiota in gut-brain axis [40].
Mounting lines of evidence demonstrate that gut microbiota dysbiosis is one of the key causative factors in stroke pathology, and the interaction between stroke and microbiota pathologies is noticeable via microbiota-gut-brain-axis. The term dysbiosis is used to indicate disruption in the balance of composition of gut microbiota towards decreased intestinal biodiversity of beneficial or commensal species and increased pathogenic bacteria species [41]. Under normal circumstances, the composition of intestinal microbiota is in a status of eubiosis, where there’s a preponderance of potentially beneficial species such as Firmicutes and Bacteroides over a very low percentage of potentially pathogenic species such as Proteobacteria. This normal balance is critical for health and homeostasis, especially in the brain, and when dysfunctional leads to the development and progression of diseases [42]. For instance, stroke leads to gut microbiota dysbiosis, and this causes dysfunctional gut-brain axis signaling that further amplified neuroinflammation and oxidative stress damage.
Several studies involving human and animal models of stroke have demonstrated gut microbiota dysbiosis involvement in the pathology of stroke. Stanley [43] examined the composition of the mucosal microbiota after stroke in a model of focal cerebral ischemia. This study found that ischemic stroke is associated with microbiota dysbiosis, as well as far-reaching and robust changes to the intestinal mucosal microbiota. Crasper [44] observed the role of bacterial translocation from the gut in post-stroke infection in an animal model of stroke. It was observed from this study that ischemic stroke results in impairment of gut permeability, as well as a marked inducement in gut dysbiosis. Stanley [45] studied whether post-stroke infection originated from commensal bacteria that normally reside in the intestinal tracts. Animal models that had post-stroke lung infection microbiota were observed, the microbes were found to originate from small intestinal microbes by more than 60%, and this was attributed to stroke-induced impairment in gut barrier that allows for intestinal microbiota to reach peripheral tissues.
Haak [46] studied the hypothesis of whether patients diagnosed with acute ischemic stroke and manifest with dysbiosis in gut microbiota composition may change the risk development of post-stroke infections following hospitalization in a case–control study. Outcome of this study revealed a drastic reduction in anaerobic gut bacteria in stroke cases, which is closely associated with post-stroke infection. Boaden [47] performed a case–control study involving patients diagnosed and screened with ischemic stroke to determine the composition of gut microbiota and its important blood metabolite trimethylamine-N-oxide. Ischemic stroke patients were found to have mark dysbiosis in gut microbiota composition with more preponderance of pathogenic microbiome and fewer commensal or beneficial microbiome. This dysbiosis was found to be correlated with the stroke severity. The blood level of trimethylamine-N-oxide was in contrast found to be significantly lower in patients with ischemic stroke. Tan [48] evaluated the gut microbiome and short-chain fatty acids spectrum, as well as their possible relation with the measure of variability in ischemic stroke severities. A mark change in composition of intestinal microbiota was observed among stroke patients when compared with control, specifically depicting deficiency in short-chain fatty acids producing bacteria which become marked with the increasing severity of stroke.
Wang [49] designed a study that sought to determine intestinal flora diversity in patients diagnosed and screened with ischemic stroke, as well as investigate whether the intestinal microflora can be used as a marker for early diagnosis of cerebral infarction. Intestinal flora composition was found to be significantly different between ischemic stroke patients in relative to the healthy control. Intestinal flora composition could be an important indicator for cerebral infarction, and due to the observed positive correlation between serum level apolipoprotein E and Gamma-proteobacteria, the serum level apolipoprotein E holds potential to predict cerebral infarction. Huang [50] carried out a study that aimed to investigate the variability in characteristics of gut microbiota among patients with acute cerebral infarction. It was revealed from this study that the abundance of three bacterial species that were abnormally higher in patients with acute cerebral ischemia in comparison to healthy controls. Yamashiro [51] observed two parameters of gut microbiota, its constituent composition, and organic acid content if they are related with ischemic stroke, and whether there is a relationship that could result in mediating biological processes such as metabolism and inflammation. Findings showed evidence of microbiota dysbiosis among the patients diagnosed with ischemic stroke, this dysbiosis was found to be associated with biological indicators of host inflammation (such as interleukin-6, high sensitivity C-reactive protein, and with white blood cell counts) and metabolism (such as acetic acid, valeric acid, and low-density lipoprotein cholesterol).
Li [52] set out a study to examine the variation in gut microbiota profile between patients diagnosed and screened with ischemic in comparison with healthy controls, and to study if such variation will be associated with the assessed clinical parameters. Patients with cerebral infarction showed dysbiosis in gut microbiota composition. This dysbiosis is related to clinical measures of stroke severity, as bacterial genera that were found to be reduced among stroke patients were negatively associated with stroke severity. Singh [53] carried out a study to determine the mechanism through which post-stroke dysbiosis links with immune response, especially the intestinal immune cells balance. Ischemic stroke was confirmed to induce dysbiosis of microbiota, and this dysbiosis proved to cause alteration in intestinal immune cells balance.
4. The role of gut microbiome in brain plasticity
Table 1 Below shows evidence about the influence of gut microbiome on brain plasticity through microbiota gut-brain axis.
Modification in microbiota gut-brain axis | Observed corresponding effect in brain plasticity process | Reference |
---|---|---|
Microbiome deficient model in the form in the germ-free (GF) mice compared with conventionally raised counterparts. | Germ-free mice show changes in electrophysiological properties of hippocampal plasticity, which is associated with dendritic signaling and long-term potentiation (LTP), indicating that absence of a microbiome alters basal synaptic efficacy, and inducibility of plasticity in the hippocampus. | [54] |
Microbiome distorted germ-free (GF) mice were compared with conventional mice. | Germ-free mice microbiome modulation with probiotics shows changes in the expression of gamma-aminobutyric acid (GABA) in the hippocampus, indicating a potential role in long-term potentiation and memory. | [55] |
The gut microbiota was altered by treatment with antibiotic or germ-free (GF) mice. | Microbiota alterations result in changes in transcriptional profiles of excitatory neurons, glia, and other cell types in the prefrontal cortex. These changes were found to have long-lasting effects on neuronal function and learning-related plasticity that subsequently regulate fear extinction behavior. | [56] |
Gut microbiota-based distorted model in germ-free (GF) mice and GF mice colonized post weaning (exGF). | Germ-free mice show difference in expression of splicing factor genes in the amygdala, which is able to partially compensate for impairments in neuronal plasticity signaling. | [57] |
Gut microbiota-based altered model in germfree (GF) mice, colonized GF mice (ex-GF) were compared with conventionally raised (CON) mice | Germ-free mice display altered transcriptional profile in genes related to cholinergic and dopaminergic neurotransmission in the amygdala (Drd2, Adora2a, Tac1, Chrnra2, and Chat) that have been implicated as crucial for neural activity, synaptic transmission, neurogenesis, and nervous system development. | [58] |
Gut microbiota-based distorted models in germ-free mice (GF; microbiota deficient from birth) were compared with conventionally colonized (CC) mice. | Germ-free mice displayed changes in volume and dendritic morphology of the amygdale, hippocampus, and pyramidal neurons, indicating the role of microbiome in neural remodeling. | [59] |
Gut microbiota-deficient animal model in the form of germ-free (GF) mice was compared with their conventionally colonized (CC) mice. | Brain-derived neurotrophic factor (BDNF) expression, a pivotal brain neurotropic molecule essential for neurogenesis and neuroplasticity was observed to be severely decreased in germ-free (GF) mice. | [60] |
A germ-free (GF) mice that have altered gut microbiota, were compared with specific pathogen-free (SPF) mice with normal gut microbiota. | A significant decrease in BDNF mRNA expression was observed in germ-free mice within the hippocampus, amygdala, and cingulate cortex. BDNF signaling mediates neural plasticity processes such as neurogenesis, dendritic arborization, and axonal sprouting. | [61] |
Gut microbiota altered model in germ-free Swiss Webster mice were compared with colonized counterparts. | Germ-free (GF) mice show distorted BDNF and c-Fos protein expression in the hippocampus. | [62] |
Table 1.
Role of gut microbiome on brain plasticity through microbiota gut-brain axis.
There exist various modalities through which gut microbiome can be modulated to complement neuroplasticity with stroke rehabilitation. Development and testing of specific interventions such as probiotics, prebiotics, dietary-based intervention, or any agent that targets particular gut microbiome metabolites will provide a potential novel strategy for gut microbiome-based intervention in stroke rehabilitation.
5. Mechanism through which microbiome-based interventions could improve stroke recovery and rehabilitation
The potentiality of microbiota gut-brain axis in the pathogenesis and therapeutics of stroke has been also promising in human studies. Guo [17] carried out a case–control study involving acute ischemic stroke patients that were treated with traditional Chinese medicine termed tanhuo decoction (THD). It was observed that THD offers neuroprotection via modulation of microbiota gut-brain axis, where it regulates several gut bacteria to lower microbial metabolites such as lipopolysaccharide (LPS) and trimethylamine N-oxide (TMAO). Zhong [63] examined evidence-based literature the effect of probiotics, as one of the specific entities within microbiome-based interventions in the management of patients with stroke. Probiotics were found to possess enormous potential for clinical applicability in promoting stroke recovery. The various mechanisms through which microbiome-based interventions could improve stroke recovery and rehabilitation are illustrated in Figure 1 below:
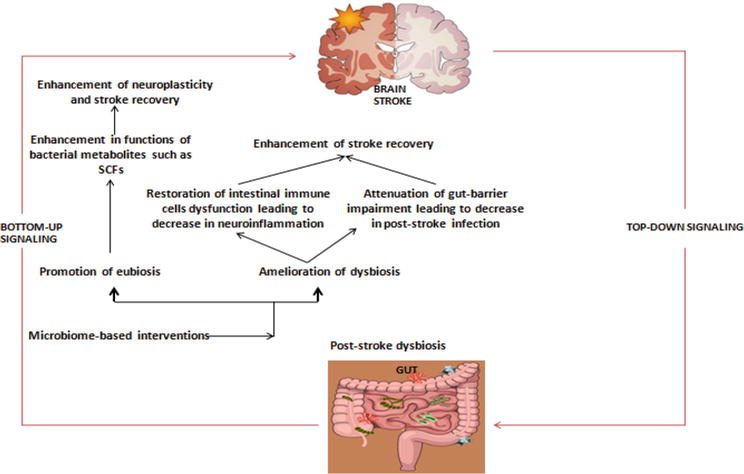
Figure 1.
Schematic illustration of various mechanisms through which microbiome-based interventions could improve stroke recovery and rehabilitation.
6. Conclusion
The role of microbiome-based modulation to mediate brain plasticity through microbiota gut-brain axis is highlighted; this could be fundamental towards translating similar phenomenon in human stroke to promote brain neuroplasticity in complement with post-stroke rehabilitation.
No comments:
Post a Comment