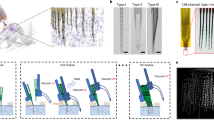
Use the labels in the right column to find what you want. Or you can go thru them one by one, there are only 29,112 posts. Searching is done in the search box in upper left corner. I blog on anything to do with stroke.DO NOT DO ANYTHING SUGGESTED HERE AS I AM NOT MEDICALLY TRAINED, YOUR DOCTOR IS, LISTEN TO THEM. BUT I BET THEY DON'T KNOW HOW TO GET YOU 100% RECOVERED. I DON'T EITHER, BUT HAVE PLENTY OF QUESTIONS FOR YOUR DOCTOR TO ANSWER.
What this blog is for:
My blog is not to help survivors recover, it is to have the 10 million yearly stroke survivors light fires underneath their doctors, stroke hospitals and stroke researchers to get stroke solved. 100% recovery. The stroke medical world is completely failing at that goal, they don't even have it as a goal. Shortly after getting out of the hospital and getting NO information on the process or protocols of stroke rehabilitation and recovery I started searching on the internet and found that no other survivor received useful information. This is an attempt to cover all stroke rehabilitation information that should be readily available to survivors so they can talk with informed knowledge to their medical staff. It lays out what needs to be done to get stroke survivors closer to 100% recovery. It's quite disgusting that this information is not available from every stroke association and doctors group.
Saturday, October 26, 2024
Neuroplasticity-related effects of vitamin D relevant to its neuroprotective effects: A narrative review
Saturday, September 28, 2024
The Effects of Prayer and Meditative Practices on Neuroplasticity
Meditation has been known for a long time to be beneficial to stroke recovery. Hasn't your competent? doctor created a protocol on it yet? So an incompetent doctor for over a decade and your stroke hospital still hasn't fired them?
meditation (60 posts to January 2012)
The Effects of Prayer and Meditative Practices on Neuroplasticity
By Masooma Hyder Baig
Throughout our lives, our brains are constantly changing and adapting to our environments. Neuroplasticity is our brain’s ability to reorganize itself by continually forming new neural connections at all life stages: good and bad. This article explores how prayer and meditative practices act as internal stimuli that impact these abilities, where research highlights the changes in brain function.
Understanding Neuroplasticity
Neuroplasticity is defined as the ability of the nervous system to change its activity in response to intrinsic or extrinsic factors by reorganizing its structure, functions, or connections (Mateos-Aparicio and Rodríguez-Moreno, 2019). This often involves the formation of new neural pathways, the strengthening of existing ones, and/or pruning of unused connections. Thus, neuroplasticity is fundamental in determining our ability to learn, memorize, and even recover from brain injuries. It enables the brain to compensate for decreased function and simultaneously maximizes the remaining function if there is damage.
Meditation and Brain changes
Meditation can refer to several mindful practices involving the individual training themselves to focus on a present moment, object, or situation, allowing them to feel peace and connected to themselves. Studies have shown that long-term meditation practices have been linked to increased cortical thickness in certain brain areas. Primarily, areas related to attention, the perception of the body’s internal conditions, and sensory processing that enable positive habits and practices in individuals. However, one should consider factors like age, sex, genetics, and health status which may also influence cortical thickness alongside the effects meditation may have (Lazar et al., 2005).
Meditation has also been linked to larger hippocampal volumes in the right hemisphere of the brain (Luders et al., 2009). The hippocampus has numerous functions, that are proven to be vital in learning and memory, emotional behavior, and spatial navigation (Dhikav and Anand, 2012). Thus, it suggests these findings explain why meditators maintain emotional stability and form positive emotions.
Prayer
Prayer encompasses many different practices which can include meditation, deep thought, and/or certain physical movements to demonstrate one’s submission to a superior being or particular way of life. Newburg’s study on the Franciscan nuns performing a contemplative prayer that involved pondering over a Bible phrase demonstrated increased frontal lobe activity and decreased parietal lobe activity (Newberg et al., 2003). The frontal lobe is associated with focused attention, emotional regulation, and decision-making (Kinaan Javed and Forshing Lui, 2019) while the parietal lobe is associated with spatial awareness and sensory perception (Vandenberghe et al., 2001). A case series study on Islamic prayer observed similar results with decreased parietal lobe activity. However, decreased frontal activity was also documented. Newburg suggested that this was due to the centering prayer of the nuns being similar to meditation, where participants were actively focused on a particular religious phrase (Newberg et al., 2015). These different practices correlate with the feeling of submission individuals may experience when in prayer, with anecdotal accounts highlighting how many feel less attached to their current surroundings and more aligned with a presence they assume to be greater than them: providing a sense of comfort during prayer.
Conclusion
To summarise, prayer and meditation both have profound effects on neuroplasticity. Regular meditation enhances cortical thickness and hippocampal volume contributing to increased attention, emotional stability, and better memory. Prayer produces similar effects to invoking different brain responses where increased frontal lobe activity may be observed. Especially in prayer practices similar to meditation that require deep focus. Other prayer practices that emphasize movement may observe decreased frontal lobe activity as one works to submit to God. Yet, these different practices reiterate the idea of relinquishing control, allowing individuals to find peace. Thus, it is evident that through prayer and meditation, one can reap both spiritual and cognitive benefits: an area of modern neuroscience proving to be abundant in further exploration.
Reference list
Thursday, September 26, 2024
The Fascinating Link between Psychedelics and Neuroplasticity
But this research says no neuroplasticity occurs. Ask your competent? doctor to clarify.
ROBUST METHODS FOR QUANTIFYING NEURONAL
MORPHOLOGY AND MOLECULAR SIGNALING REVEAL THAT
PSYCHEDELICS DO NOT INDUCE NEUROPLASTICITY
March 2024
The latest here:
The Fascinating Link between Psychedelics and Neuroplasticity
In recent years, the scientific community has started to unveil one of the most intriguing possibilities of treatment of modern times: the use of psychedelics to induce or promote neuroplasticity for therapeutic purposes. This exploration is not a mere revisiting of the countercultural relics of the 1960s; rather, it aims at reappraising a journey into the depth of human mind to seek answers to some of the most pressing mental health challenges [1]. Psychedelics, such as lysergic acid diethylamide, mescaline, psilocybin (found in magic mushrooms), and N-dimethyltryptamine (DMT) (present in ayahuasca), are known for their relevant effects on perception, thought, and emotion, often leading to mystical experiences. Once sidelined their detrimental effects, however, these compounds are increasingly recognized for their potential to rewire the brain, especially when it is resistant to traditional treatments [2].
From a neurobiological perspective, these substances are linked to the well-documented hallucinogenic effects, mostly involving the activity of the fronto-cortical network. Recent insights, however, suggest that psychedelics may also promote structural plasticity and neuronal growth within the fronto-cortical-limbic circuits, likely through an increased levels of the Brain-Derived Neurotrophic Factor (BDNF) [3], a vital protein for neuronal growth and survival. This effect, known as “psychoplastogen”, fosters neuroplastic changes that are pivotal for synaptic, structural, and behavioral adaptations, both in normal and pathological conditions. Neuroplasticity, i.e., the brain’s ability to reorganize itself by generating new neural connections, is vital for learning, memory, and sleep, among others, as well as for the recovery from different types of brain injury. Apart from pure motor tasks, indeed, neural plasticity plays a crucial role also in cognitive functioning and mental health [4]. Accordingly, impaired or maladaptive neuroplasticity has been linked to several neurological and neuropsychiatric disorders, such as depression and age-related cognitive decline [5]. Psychedelics might mitigate synaptic deficit, neuronal degeneration, and the loss of network connectivity, which are all linked to chronic stress, sustained neuroinflammation, depression, and treatment resistance [6]. This potential for neuroplasticity and recovery is one of the reasons underlying the exploration of psychedelics as new treatments for mental and cognitive disorders.
Pharmacologically, psychedelics act on the serotonin 5-HT2A receptor (5-HT2AR), which are pivotal in regulating mood, cognition, and perception. This activation leads to specific changes in neurotransmitter signaling, particularly within the serotoninergic system, which is involved not only in mood regulation but also in cognition. Accordingly, it is known that some of the key cognitive functions where serotonin exerts a crucial role include short-term and long-term memory, decision making, attention, and executive function, as well as learning and social behavior [3]. However, the exact mechanism through which the 5-HT2AR activation influences neuronal growth remains largely unclear. In this scenario, the neuroplastic effects of psychedelics are believed to be mediated by several signaling pathways, including the tropomyosin receptor kinase B (TrkB), the mammalian target of rapamycin (mTOR), and the α
-amino-3-hydroxy-5-methyl-4-isoxazolepropionic acid (AMPA) receptor glutamate signaling. Overall, these pathways coordinate the rapid and sustained enhancement of synaptic and structural neuronal remodeling, which seems to contribute to the clinical effect of psychedelics in mental health disorders, probably through the counteract of synaptic deficit and neuronal loss [3, 7].
Interestingly, human research using neuroimaging and post-mortem studies, as well as some animal models, have revealed that psychedelics can exert an anti-apoptotic effect on neurons in the prefrontal cortex (PFC) [8, 9, 10, 11, 12, 13]. This degeneration is typically exacerbated by stress, suggesting that psychedelics might counteract such detrimental effects through their neuroplastic properties. Furthermore, these substances stimulate the growth of new neural connections and enhance communication across different brain regions [7]. The activation of this pathway also initiates a cascade of neurobiological events eventually leading, among others, to an increased expression of BNDF. In particular, DMT has been associated with an increase in peripheral BDNF levels, highlighting a clear impact of psychedelics on this important neurotrophic factor [6, 14].
The downstream effect of psychedelics involves the upregulation of transcription and translation of synaptic proteins within the PFC and the hippocampus. Notably, these substances are able to induce long-lasting alterations in synaptic function and morphology also at the level of pyramidal neurons. As such, psychedelics have been linked to a temporary rise in cortical glutamate release and prolonged activation of excitatory neurotransmission in pyramidal neurons, primarily within the PFC [6], thus suggesting a role also at premotor and motor level.
Finally, psychedelics significantly impact brain connectivity, enhancing overall brain interactions and linking regions that normally would not communicate directly. This increased connectivity can lead to unique experiences, such as synesthesia, where senses overlap (e.g., seeing sounds), and intense perceptions (more than normal). Specifically, psychedelics reduce the activity in the Default Mode Network (DMN), which is associated with self-referential thoughts and the ego awareness; these changes can also include disruptions in thalamo-cortical connectivity, thus reducing the filtering of sensory inputs and intensifying sensory experiences. These alterations in functional connectivity are of particular interest for therapeutic applications, offering potential benefits for treating specific mental health issues, such as depression, post-traumatic stress disorder, and addiction disorders, by “resetting” dysfunctional brain networks [9]. The ongoing research into these effects holds promise for new insights into consciousness and mental health treatment [3]. A schematic summary of perspectives/strenghts and challenges/limitations associated with psychedelic therapy are detailed in the below-reported table (see Table 1).
Aspect to be considered | Perspectives/Strenghts | Challenges/Limitations |
---|---|---|
Mental Health | Potential to treat resistant mental health disorders including depression, post-traumatic stress disorders, and addiction. | Variability in individual responses; potential for adverse psychological effects. |
Neuroplasticity and Brain Connectivity | Promotes structural plasticity, neuronal growth, and enhances brain interactions, potentially leading to improved cognitive and emotional processing. | Mechanisms of action are not fully understood; changes in connectivity might lead to unpredictable outcomes. |
Regulatory and Safety | Growing interest may lead to the development of new therapeutic protocols and guidelines/consensus statements. | Lack of comprehensive regulatory frameworks; safety concerns due to powerful and possibly unpredictable effects. |
Research and Clinical Trials | Ongoing research holds promises for new insights and treatments. | Need for more rigorous and extensive clinical trials to establish efficacy and safety profiles. |
Socio-cultural Acceptance | Increased acceptance may integrate psychedelics into mainstream therapeutic options. | Socio-cultural stigma and legal barriers still present may significantly limit a widespread acceptance and a diffuse adoption. |
Concluding, the exploration of the complex but intriguing connections between psychedelics and neuroplasticity opens innovative avenues of research in the understanding and potentials of these molecules in mental health disorders. Although preliminary data seem to be promising, further research is needed to fully disentangle the mechanisms of action, optimize therapeutic protocols, and ensure a safe use of these substances [15]. Currently, however, integrating psychedelics into mainstream therapy faces significant clinical and socio-cultural challenges. Of course, safety always comes first, since the powerful nature of these substances are able to induce profound changes which, in the absence of solid scientific evidence and proper therapeutic guidance, might lead to adverse events and undesiderable outcomes. Comprehensive regulatory frameworks, rigorous clinical trials, and accurate training for operators are essential steps of this challenging but also fascinating path of modern neuropsychiatry.
Tuesday, September 3, 2024
Longitudinal study of stroke-induced neuroplasticity using imaging and high-density neural recording
But your competent? doctor would already have started listening to brain signals using one of these already to make neuroplasticity repeatable on demand. Add sarcasm tag here.
1. Use nanowires to listen in on single neurons
2. Or lay a grid across the cortex
to listen in.
But we have NO stroke leaders, nothing will get done until we get survivors in charge.
Leaders solve problems, they don't run away from them. We have NO leaders in stroke!
Longitudinal study of stroke-induced neuroplasticity using imaging and high-density neural recording
Abstract
The brain has a remarkable ability to undergo spontaneous self-repair in response to an injury or a cortical lesion. This dynamic and lasting restorative process involves a diverse array of neuroplastic mechanisms that are time and location dependent. Lesion-induced neuroplasticity is believed to involve neighboring intact neurons assuming the functional roles of the damaged ones, altering the brain’s activation map. Despite extensive research, there is still a lack of definitive evidence regarding neurons changing their functional response, leading to debates about the exact cellular mechanism of neuroplasticity. Conclusive evidence supporting or refuting functional remapping after stroke requires direct measurements and longitudinal tracking of neural activity at a single-neuron resolution and over chronic periods.
In this work, we employed multi-electrode arrays (NanoElectronic Threads) to record and longitudinally track both the sensory-evoked single-neuron spiking dynamics and population activity with high spatial specificity after a small-scale optically targeted stroke. Our multimodal measurement combined simultaneous laser speckle contrast imaging and hyperspectral reflectance spectroscopy, together with spatially distributed intracortical neural recordings. We found that while hemodynamic activation shifted following the cortical lesion, it no longer correlated with electrical neural activity. Direct neural recording showed a sustained suppression of evoked spiking activity near the lesioned infarct while an enhancement in the more distant cortical regions. Longitudinal tracking of individual neurons uncovered heterogeneous responses underlying the enhanced activity. We observed a distinct subset of neurons that demonstrated a significant upregulation in their sensory-evoked spiking activity which exhibited a stronger correlation with the overall population activity within the local cortical network, suggesting a potential restorative mechanism in response to the lesion. Contrary to the prevailing hypothesis of population-level changes in the peri-infarct region, our findings provide compelling evidence against this notion. Instead, our data reveal that lesion-induced plasticity at the single neuron level is manifested as a selective potentiation of pre-existing functional neurons.
Advisor
Degree
Type
Keywords
Citable link to this page
Friday, July 5, 2024
Neuroplasticity and Rehabilitation: Harnessing Brain Plasticity for Stroke Recovery and Functional Improvement
Still nothing on EXACTLY how to get neuroplasticity working.
Neuroplasticity is often mentioned as to how survivors will recover, that's a useless statement because no one has identified the signals occurring between neurons that tell a neighboring neuron to drop its' current function and take up a neighboring neurons function. Until that is identified neuroplasticity will never be repeatable on demand.
Neuroplasticity and Rehabilitation: Harnessing Brain Plasticity for Stroke
Recovery and Functional Improvement
Psychiatrist at National Institute of Mental
Health and Neurosciences (NIMHANS),
Chennai
DOI: https://doi.org/10.36676/urr.v11.i3.1287
Accepted: 10/05/2024 Published: 30/06/2024 * Corresponding author
Abstract:
neuroplasticity and its application in stroke rehabilitation. Stroke remains a leading cause of
disability worldwide, often resulting in motor, sensory, and cognitive impairments.
Neuroplasticity, the brain's ability to reorganize and adapt in response to experience and
injury, offers promising avenues for recovery. This review discusses key principles of
neuroplasticity and explores various rehabilitation strategies aimed at harnessing its potential
for stroke recovery. Topics covered include early intervention, task-specific training, intensity
and repetition, constraint-induced movement therapy, multimodal approaches, environmental
enrichment, and neurostimulation techniques. Additionally, the paper discusses emerging
research directions and challenges in optimizing neuroplasticity-based rehabilitation
approaches. Understanding the role of neuroplasticity in stroke recovery can inform the
development of more effective rehabilitation interventions and improve outcomes for
individuals affected by stroke.
Keywords: neuroplasticity, stroke rehabilitation, motor recovery, sensory recovery, cognitive
rehabilitation
Introduction
Stroke remains one of the leading causes of disability globally, presenting significant
challenges to individuals, families, and healthcare systems. Every year, millions of people
worldwide experience stroke, resulting in a wide range of physical, cognitive, and emotional
impairments. While advancements in acute stroke care have improved survival rates, the need
for effective rehabilitation strategies to promote recovery and improve functional outcomes is
paramount. Central to the quest for enhanced stroke rehabilitation is the concept of
neuroplasticity. Neuroplasticity refers to the brain's remarkable ability to reorganize its
structure and function in response to experiences, learning, and injury. This phenomenon
underlies the brain's capacity to adapt following stroke, offering hope for recovery even in the
face of significant neurological damage.
Understanding the mechanisms and principles of neuroplasticity is crucial for developing and
optimizing rehabilitation interventions aimed at facilitating stroke recovery. By harnessing the
SHODH SAGAR®
Universal Research Reports
ISSN: 2348-5612 | Vol. 11 No. 3 (2024): Special Issue: Advances in Medical Research | June 2024
51
© 2024 Published by Shodh Sagar. This is a Gold Open Access arƟcle distributed under the terms of the CreaƟve Commons License
[CC BY NC 4.0] and is available on hƩps://urr.shodhsagar.com
brain's inherent plasticity, rehabilitation strategies can promote neural rewiring, functional
reorganization, and ultimately, improved outcomes for individuals affected by stroke.
Neuroplasticity: Foundations and Mechanisms
Neuroplasticity, the brain's remarkable ability to reorganize and adapt in response to
experience, injury, or environmental changes, lies at the heart of stroke rehabilitation.
Understanding the foundational principles and underlying mechanisms of neuroplasticity is
essential for designing effective rehabilitation strategies aimed at promoting recovery
following stroke. Neuroplasticity encompasses a broad spectrum of adaptive changes in the
brain, ranging from synaptic modifications at the cellular level to large-scale reorganization of
neural circuits. It reflects the brain's intrinsic capacity for structural and functional remodelling
throughout life. The mechanisms underlying neuroplasticity involve complex interactions
between neurons, glial cells, neurotransmitters, and molecular signalling pathways. At the
cellular level, synaptic plasticity plays a central role in encoding and consolidating learning
and memory. Long-term potentiation (LTP) and long-term depression (LTD) are two well-
studied forms of synaptic plasticity that contribute to experience-dependent changes in neural
connectivity. On a macroscopic scale, cortical remapping refers to the reorganization of cortical
representations in response to sensory or motor input. Following stroke, cortical areas adjacent
to the lesion may undergo functional reorganization to compensate for lost functions, a
phenomenon known as diaschisis. Additionally, axonal sprouting and dendritic remodelling
can facilitate the formation of new connections and neural pathways, contributing to functional
recovery. In the context of stroke recovery, several forms of neuroplasticity are particularly
relevant, including reactive plasticity, compensatory plasticity, restorative plasticity, and
experience-dependent plasticity. Understanding the interplay between these different forms of
neuroplasticity is critical for tailoring rehabilitation interventions to individual patients' needs
and optimizing outcomes following stroke.
Friday, June 21, 2024
Promoting and Delivering Physical Activity Interventions After Stroke: A Global Educational Needs Survey of Health Professionals
I'd suggest filling this out from a survivor perspective. We need EXACT 100% RECOVERY PROTOCOLS! Guidelines are fucking worthless.
We’re creating an educational resource for #stroke clinicians to support people to be #physicallyactive after stroke.
Saturday, June 8, 2024
Months-long tracking of neuronal ensembles spanning multiple brain areas with Ultra-Flexible Tentacle Electrodes
This is where stroke leaders would look at this
and mutter to themselves; 'We could use this to detect brain signals
occurring during stroke recovery; neurogenesis and neuroplasticity, and
thus figure out how to make those recovery signals repeatable'. But we have to do
human testing in stroke survivors first.
But the leaders would already have started listening to brain signals using one of these already. Add sarcasm tag here.
1. Use nanowires to listen in on single neurons
2. Or lay a grid across the cortex
to listen in.
But we have NO stroke leaders, nothing will get done until we get survivors in charge.
Leaders solve problems, they don't run away from them.
Ask your competent? doctor if this is better for tracking neuronal signals than these other ones?
The latest here:
Months-long tracking of neuronal ensembles spanning multiple brain areas with Ultra-Flexible Tentacle Electrodes
- Tansel Baran Yasar,
- Peter Gombkoto,
- Alexei L. Vyssotski,
- Angeliki D. Vavladeli,
- Christopher M. Lewis,
- Bifeng Wu,
- Linus Meienberg,
- Valter Lundegardh,
- Fritjof Helmchen,
- Wolfger von der Behrens &
- Mehmet Fatih Yanik
Nature Communications volume 15, Article number: 4822 (2024) Cite this article
-
177 Accesses
-
3 Altmetric
Abstract
We introduce Ultra-Flexible Tentacle Electrodes (UFTEs), packing many independent fibers with the smallest possible footprint without limitation in recording depth using a combination of mechanical and chemical tethering for insertion. We demonstrate a scheme to implant UFTEs simultaneously into many brain areas at arbitrary locations without angle-of-insertion limitations, and a 512-channel wireless logger. Immunostaining reveals no detectable chronic tissue damage even after several months. Mean spike signal-to-noise ratios are 1.5-3x compared to the state-of-the-art, while the highest signal-to-noise ratios reach 89, and average cortical unit yields are ~1.75/channel. UFTEs can track the same neurons across sessions for at least 10 months (longest duration tested). We tracked inter- and intra-areal neuronal ensembles (neurons repeatedly co-activated within 25 ms) simultaneously from hippocampus, retrosplenial cortex, and medial prefrontal cortex in freely moving rodents. Average ensemble lifetimes were shorter than the durations over which we can track individual neurons. We identify two distinct classes of ensembles. Those tuned to sharp-wave ripples display the shortest lifetimes, and the ensemble members are mostly hippocampal. Yet, inter-areal ensembles with members from both hippocampus and cortex have weak tuning to sharp wave ripples, and some have unusual months-long lifetimes. Such inter-areal ensembles occasionally remain inactive for weeks before re-emerging.
Similar content being viewed by others
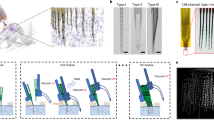
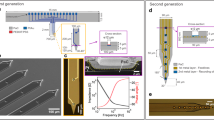
Minimally-invasive insertion strategy and in vivo evaluation of multi-shank flexible intracortical probes
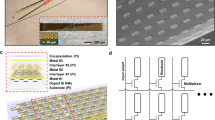
A soft, high-density neuroelectronic array
Introduction
Complex behaviors such as perception, decision-making, and learning involve the interaction of many brain areas1,2. Techniques to perform long-term recordings of brain activity at single-cell resolution simultaneously from distributed brain areas are essential to investigate the coordinated brain dynamics underlying the learning and execution of such behaviors. Such high-resolution recordings can also improve our understanding of brain disorders and enable the development of new diagnostics and treatments that would be otherwise combinatorially impossible to discover using behavioral readouts alone3. However, currently available technologies for such recordings are limited, both in terms of their long-term stability and their applicability to multi-areal recordings. Although optical methods enable stable recordings of single-cell activity4, they cannot reach deep brain areas such as the hippocampus or thalamus without damaging the intermediate brain areas and require compromises between the number of cells recorded and temporal resolution5. They also require genetic modifications or the delivery of fluorescent indicators to neurons, making them impractical to use in the human brain.
Extracellular electrode arrays, on the other hand, are typically fabricated on stiff materials and with large footprints that do not comply with the brain tissue, leading to glial encapsulation of the electrode arrays, loss of single units, low unit yields, drifts in recordings, and the inability to track single units across sessions6,7,8,9,10, unless ultra-high-density contacts are used to compensate for drifts and instabilities11,12. Flexible polymer electrode arrays have emerged as alternatives to stiff probes, offering superior biocompatibility, adaptability to the mechanics of the brain tissue, and stability of recordings13,14,15,16,17. However, recording from multiple brain areas with high-density flexible electrode arrays at cellular scale (<10 μm) without limitations on implantation depth while minimizing the implants’ footprints still remains a major challenge. Integrating more recording contacts on polymer shanks requires them to be wider, which leads to cutting through more neuronal structures during insertion (Fig. 1a). While this can be mitigated by distributing the recording contacts into independent tiny electrode fibers, inserting many such fibers simultaneously has been challenging due to their high flexibility and the shortcomings of the chemical glues to keep them together (Fig. 1b). For instance, polyethylene glycol, a water-soluble material commonly used for tethering electrode arrays to shuttles, can be dissolved in seconds inside the brain or even near the brain surface due to the humidity. Achieving stiffness or stability for insertion by using even more adhesive alone leads to larger insertion footprints. If, instead, a stiff insertion shuttle is used to achieve stiffness while holding together all the fibers and shuttle using a strong glue, the electrode fibers often get pulled out during retraction of the stiff insertion shuttle (Fig. 1b).
a Drawn-to-scale comparison of the geometries of a rigid silicon probe, flexible planar shank probe, neural mesh, and UFTE (from left to right) with reference to the soma and dendrites of surrounding neurons. Zoomed insets (bottom) show the impact of different probe geometries on neuronal processes after insertion into the brain. b Potential failures during the insertion of ultra-flexible electrode arrays coupled to a stiff shuttle purely by chemical tethering (left). The electrode fibers can separate prematurely from the stiff shuttle if the coating dissolves too fast (middle), or they can get stuck to the shuttle and be pulled out of the brain during shuttle retraction if the coating dissolves too slowly (right). c Delivery of the UFTE bundle into the brain, where the bundle is inserted into the brain with the help of a tungsten shuttle, the shuttle is retracted, and the silk coating is dissolved, leaving the electrode fibers independent from each other. d UFTE 3.5 months post-implantation. (i) UFTE bundle (red) shown with stained neurons (magenta) and the microglia (cyan). (ii) Loop of UFTE bundle in the same brain slice. Scale bars are 100 μm.
To overcome these issues, we developed Ultra-Flexible Tentacle Electrode (UFTE) arrays, which provide stable recordings of single units with exceptionally high mean signal-to-noise ratios (SNRs) of single-unit spikes, 1.5–3 times larger than state-of-the-art flexible electrode arrays15,16, with some mean single-unit SNRs as large as 89. We demonstrate that UFTEs can be inserted at least 6.5 mm deep from the dorsal surface while achieving high single-unit yields per recording contact. We also developed a technique to insert UFTEs simultaneously into many brain areas at arbitrary locations without any depth and angle-of-insertion limitations (Fig. 2d, e) to vastly simplify distributed recordings with UFTEs.
More at link.
Thursday, May 30, 2024
Integration of music-based game approaches with wearable devices for hand neurorehabilitation: a narrative review
I bet your competent? doctor still hasn't created a music protocol for your recovery! And you're still seeing them?
music (94 posts back to March 2011)
music therapy (53 posts back to October 2014)
musical training (13 posts back to June 2014)
Integration of music-based game approaches with wearable devices for hand neurorehabilitation: a narrative review
Journal of NeuroEngineering and Rehabilitation volume 21, Article number: 89 (2024) Cite this article
Abstract
Background
Restoring hand functionality is critical for fostering independence in individuals with neurological disorders. Various therapeutic approaches have emerged to address motor function restoration, with music-based therapies demonstrating notable advantages in enhancing neuroplasticity, an integral component of neurorehabilitation. Despite the positive effects observed, there remains a gap in the literature regarding implementing music treatments in neurorehabilitation, such as Neurologic Music Therapy (NMT), especially in conjunction with emerging fields like wearable devices and game-based therapies.
Methods
A literature search was conducted in various databases, including PubMed, Scopus, IEEE Xplore, and ACM Digital Library. The search was performed using a literature search methodology based on keywords. Information collected from the studies pertained to the approach used in music therapy, the design of the video games, and the types of wearable devices utilized.
Results
A total of 158 articles were found, including 39 from PubMed, 34 from IEEE Xplore, 48 from Scopus, 37 from ACM Digital Library, and 35 from other sources. Duplicate entries, of which there were 41, were eliminated. In the first screening phase, 152 papers were screened for title and abstract. Subsequently, 89 articles were removed if they contained at least one exclusion criterion. Sixteen studies were considered after 63 papers had their full texts verified.
Conclusions
The convergence of NMT with emerging fields, such as gamification and wearable devices designed for hand functionality, not only expands therapeutic horizons but also lays the groundwork for innovative, personalized approaches to neurorehabilitation. However, challenges persist in effectively incorporating NMT into rehabilitation programs, potentially hindering its effectiveness.
Introduction
Neurological disorders are a group of heterogeneous diseases, some of which contribute to gait, balance, and strength problems that result in a lower quality of life. They affect nearly one billion people globally and comprise 6.3% of the worldwide disease burden [1]. Representative cases in this group are stroke, multiple sclerosis, cerebral palsy, spinal cord injury (SCI), and Parkinson’s disease (PD) [2]. These disorders can lead to significant deterioration in hand function, one of the most complex anatomical structures, and crucial in the human capacity for executing activities of daily living [3]. Particularly in individuals with these conditions, due to the significant deterioration in hand function, the ability to reach, grasp, release, and move objects effectively is frequently affected as a result of impairments of upper extremity function: reduced muscle strength, sensory loss, increased muscle stiffness, and a lack of motor control [4].
One of the primary methods of recovery after a neurological injury is neuroplasticity [5]. This phenomenon is defined as the capacity of the human brain to adapt and reconfigure its neuronal connections in response to diverse experiences [6]. When changes in the brain are connected to dysfunctional outcomes for the individual, it is termed maladaptive neural plasticity [7], such as neurological disorders. Conversely, when alterations in the brain are correlated with enhancements in an individual’s behavioral capabilities, this phenomenon is termed adaptive neural plasticity [7]. Some examples of the appearance of adaptive neural plasticity are during brain development [8] and motor skills learning [9]. As a result, ongoing research is dedicated to devising innovative treatments that can enhance neuroplasticity to rehabilitate patients with neurological disorders.
Evidence supports the effectiveness of including intensive, repetitive, challenging, and task-specific practices in interventions aimed at fostering neuroplasticity(But you have NO CLUE on how to make neuroplasticity repeatable on demsnd!) and augmenting sensorimotor recovery [10,11,12,13]. Furthermore, Petzinger et al. [12] underscore the significance of cognitive engagement as another critical component for enhancing plasticity. These authors suggest that cognitive engagement might be improved by feedback, attentional demand through cueing, and motivation. Cueing is described as utilizing external temporal or spatial stimuli to aid in initiating and sustaining movement [14].
Standard neurorehabilitation techniques for upper limb movement primarily depend on physical therapy [15]. This therapy involves targeted exercises designed to restore the functioning of the affected portion of the motor system [16]. Unfortunately, traditional occupational therapy in its current form is a process that patients dislike going through [17], as it proves difficult for them to sustain interest in repetitive exercise routines while simultaneously focusing on the precision of their movements (e.g., speed, precision, fluidity, and posture) [18, 19] and assumes greater significance considering that a patient’s attitude during physical therapy sessions is closely related to their compliance and success [20]. Children are significantly more problematic in this area since it is challenging to maintain their motivation throughout protracted therapy sessions [21]. Moreover, heightened patient motivation in their ultimate objective is a crucial facet of neuroplasticity [22]. Therefore, based on the reviewed literature, it can be inferred that effective strategies for improving neuroplasticity in neurorehabilitation should involve elements such as intensity, repetition, challenges, task-specificity, and cognitive engagement, among others.
Consequently, in upper limb neurorehabilitation, many therapy options have emerged. Lin et al. [23] realized a review of experimented training programs designed to enhance motor recovery following a stroke; these include Constraint-Induced Movement Therapy, Electromyography biofeedback, Motor imagery therapy, Robot-assisted training, Virtual reality or gaming, etc. These treatment options must incorporate the abovementioned components to promote neuroplasticity in patients.
In the quest to provide hand neurorehabilitation with intensive, repetitive, and task-specificity practices for the patient, taking into account their limitations and strengths, it is of paramount importance to assess hand functionality during therapies, as it enables the identification of changes indicating neurological decline or the tracking of responses to treatments [24]. In this regard, motion capture devices gain greater significance. Camera-based systems and keyboard-based methods, such as the Musical Instrument Digital Interface (MIDI), are widely used for movement assessment in rehabilitation. Notable studies include those employing the leap motion controller, such as a 2019 study on PD by Fernández-González et al. [25] and a study on stroke by Shah et al. [26]. Additionally, studies have used keyboards by Altenmüller in 2009 [27] and Villeneuve in 2013 [28]. However, wearable devices distinguish themselves by assessing user movement and providing assistance during rehabilitation. For instance, wearable robotic devices outperform traditional therapy due to their ability to provide a higher number of repetitions in each session, objectively assess the patient’s performance, reduce the physical strain on therapists, and enable the monitoring of the patient’s active participation in the training regimen [29]. In the same way, non-robotic wearable devices, such as data gloves, are effective instruments for tracking hand movements and evaluating hand functionality within hand rehabilitation systems [30].
One practical approach to enhancing cognitive engagement involves the use of auditory cueing. One emerging rehabilitation process that uses auditory cues is music therapy, specifically neurological music therapy (NMT) [31]. NMT is one of the few clinical interventions utilizing music as a primary rehabilitative stimulus to evoke diverse brain and motor responses [32]. Additionally, evidence suggests that musical treatments provide a therapeutic strategy for recovering functional capacities in the upper extremities of individuals with neurological disorders [33]. This is accentuated by its reliance on a research-based system of standardized clinical techniques for sensorimotor, speech/language, and cognitive training [34].
Thaut and Hoemberg [35] have classified NMT into twenty techniques, from which three address motor rehabilitation: Rhythmic Auditory Stimulation (RAS), Patterned Sensory Enhancement (PSE), and Therapeutic Instrumental Music Performance (TIMP). RAS enhances motor control by applying rhythmic sensory stimulation in rehabilitating movements with inherent biological rhythmicity, such as gait [35]. Although RAS has been extensively studied for gait neurorehabilitation, especially on PD [36], it is also applied to upper limb rehabilitation. Ghai et al. [37] realized a systematic review and meta-analysis to analyze the effects of rhythmic auditory cueing on arm function recovery post-stroke, in which beneficial effects on the Fugl Meyer test, Action reach arm test and Wolf motor time test were reported. PSE is utilized for movements that do not inherently follow a rhythm, such as typical arm and hand motions. Besides employing rhythm and timing as cues for movement, like RAS, PSE utilizes intricate patterned structures in music to organize multiple smaller motions to accomplish a more extensive sequence of movements [35]. An investigation led by Wang et al. [38] within a home-based program employing PSE reveals significant enhancements in the gross motor capacity of children with cerebral palsy. Likewise, in a recent study by Fan et al. [39], individuals with PD demonstrated enhanced speed and functionality in upper-limb movements by integrating PSE. Finally, TIMP uses musical instruments to assist patients in regaining effective movement patterns and exercising compromised motor function [35]. According to Pascual-Leone, playing a musical instrument demands extensive procedural and motor learning that results in the plastic reorganization of the human brain, which further supports the potential of music therapy in rehabilitation [40]. A systematic review performed by Yang et al. in 2022 summarizes the effect of NMT in patients with cerebral palsy. It suggests the effect of TIMP in enhancing both gross and fine motor skills, with a particular focus on improving hand function and the power associated with piano key pressing [41].
A growing aspect highlighted in Lin et al.’s [23] review of neurological rehabilitation is the incorporation of gaming, specifically the integration of gamification. Gamification is characterized as implementing game-related components in settings that extend beyond traditional gaming contexts [42]. It is suggested that incorporating gamification into neurorehabilitation has the potential to tackle various challenges related to the implementation of intensive, engaging, and cost-effective therapeutic exercises [43], even promoting motivation [44]. Games introduce challenges to patients, transforming rehabilitation into a dynamic and appealing journey [45]. Numerous research groups have advocated integrating video games as a supplementary tool alongside traditional neurorehabilitation therapy [46].
In summary, there is a need to develop innovative approaches for delivering therapeutic exercises, especially for hand neurorehabilitation. Gathering evidence in rehabilitating neurological disorders and enhancing neuroplasticity and patient adherence underscores that interventions integrating repetitive, intensive, challenging, and motivational tasks are highly likely to improve functional recovery. For this reason, this narrative review aims to investigate the effectiveness of music and game-based approaches, hand functionality assessment, and assistance by wearable devices in achieving neuroplasticity for successful hand neurorehabilitation.