They never say if this will be able to provide 100% recovery in the time you'll be able to use this in the hospital.
Mechanical Design and Analysis of the End-Effector Finger Rehabilitation Robot (EFRR) for Stroke Patients
1 Parallel Robot and Mechatronic System Laboratory of Hebei Province, Yanshan University, Qinhuangdao 066004, China
2 Key Laboratory of Advanced Forging &
Stamping Technology and Science of Ministry of Education, Yanshan
University, Qinhuangdao 066000, China
3 Academy for Engineering & Technology, Fudan University, Shanghai 200433, China
4 State Key Laboratory of Robotics and System, Harbin Institute of Technology, Harbin 150080, China
5 College of Arts & Design, Yanshan University, Qinhuangdao 066004, China
*
Author to whom correspondence should be addressed.
Academic Editor: Giovanni Legnani
Machines 2021, 9(6), 110; https://doi.org/10.3390/machines9060110
Received: 26 April 2021 / Revised: 19 May 2021 / Accepted: 24 May 2021 / Published: 26 May 2021
(This article belongs to the Special Issue Design and Control of Advanced Mechatronics Systems)
Most existing finger rehabilitation robots are structurally complex and
cannot be adapted to multiple work conditions, such as clinical and
home. In addition, there is a lack of attention to active
adduction/abduction (A/A) movement, which prevents stroke patients from
opening the joint in time and affects the rehabilitation process. In
this paper, an end-effector finger rehabilitation robot (EFRR) with
active A/A motion that can be applied to a variety of applications is
proposed. First, the natural movement curve of the finger is analyzed,
which is the basis of the mechanism design. Based on the working
principle of the cam mechanism, the flexion/extension (F/E) movement
module is designed and the details used to ensure the safety and
reliability of the device are introduced. Then, a novel A/A movement
module is proposed, using the components that can easily individualized
design to achieve active A/A motion only by one single motor, which
makes up for the shortcomings of the existing devices. As for the
control system, a fuzzy proportional-derivative (PD) adaptive impedance
control strategy based on the position information is proposed, which
can make the device more compliant, avoid secondary injuries caused by
excessive muscle tension, and protect the fingers effectively. Finally,
some preliminary experiments of the prototype are reported, and the
results shows that the EFRR has good performance, which lays the
foundation for future work.
Keywords:
finger rehabilitation robot; mechanical design; adduction/abduction movement module; adaptive impedance control; preliminary tests
1. Introduction
According
to the World Health Organization (WHO), there are more than 15 million
people around the world suffer strokes every year, and about 5 million
of them are permanently disabled. Moreover, the global lifetime risk of
stroke from the age of 25 years onward has increased from 22.8% in 1990
to 24.9% in 2016. Stroke is still the main cause of death worldwide [1,2,3].
Stroke causes damage to the nervous system, and can cause patients to
lose part or all of their ability for activities of daily living (ADL),
which brings a heavy burden to the family and society. Currently, the
functional impairment of the fingers poststroke is rarely considered
life-threatening. Together with the limited resources (time, cost,
number of the caregivers and equipment etc.), it rates low on the
priority of rehabilitation tasks [4,5,6].
In some ways, this increases the number of people with finger injury
sequelae. Therefore, research on finger rehabilitation after stroke
should be given more attention.
Rehabilitation
robots can assist poststroke patients with continuous, repetitive
training with a standardized process, reducing the workload of
therapists and providing more clinical options for patients. Hand
rehabilitation robots can be divided into end-effector devices,
exoskeletons and glove type in terms of wearing form [7]. Among all the end-effector devices, Amadeo [8,9] is the most commercially successful hand rehabilitation robot available. HandCARE [10]
adopts ropes to fix fingers, and a clutch system is designed to allow
all the fingers to be driven by only one motor. Rutgers Master II [11]
is a four-degree-of-freedom pneumatically driven finger rehabilitation
device which has been clinically tested. It achieves active
flexion/extension (F/E) motion for four fingers, and the maximum output
force can reach 16.4 N. Reha-Digit [12]
is a passive rehabilitation device: patients need to put the fingers
into four plastic roller sets during the training process. SAFE [13]
drives the patient’s fingertips to do rehabilitation exercises by using
rigid connecting rod structures. In addition, some universities have
conducted researches on end-effector finger rehabilitation devices [14,15]. As for the exoskeletons, the robot developed by Gifu University [16]
controls all of the fingers independently by arranging side-by-side
dual motors on the back of the hand. The researchers at the University
of Texas at Austin [17,18]
applied the series elastic actuator (SEA) to finger rehabilitation.
Their device uses Bowden cables to transmit power and obtains joint
information through angle sensors, and it is lightweight and
easy-to-wear by moving the motors outside of the back of the hand. The
rehabilitation robotic exoskeleton hand [19]
realizes finger F/E training through two worm gears, and passive pins
are set to achieve adduction/abduction(A/A) motion simultaneously and a
virtual reality system has been developed for rehabilitation scenarios.
The Powered Finger–Thumb Wearable Hand Exoskeleton [20]
adopts an under-driven cord control form and designed a self-alignment
mechanism that prevents misalignment for the joints between the human
and machine, besides, parallel mechanism has been attempt to applied on
the hand rehabilitation [21].
The glove-type devices have developed significantly in recent years due
to their good adaptability. Their driving form includes pneumatic [22,23], cord drive [24], layered reed drive [25,26,27] and so on.
Force-based control is one of the control strategies for finger rehabilitation robots [28]. Cheng et al. [29]
proposed a controller combing the iterative learning control (ILC) and
the active disturbance rejection control (ADRC) to adapt the repeating
training manner and overcome the external interference in a wearable
hand rehabilitation robot. Park et al. [30]
used proportional-integral-derivative (PID) control to design a control
strategy capable of automatically switching between position and force
control. Chiri et al. [31] utilized the PID control strategy to compensate for the external forces exerted by the patient on the robot. Huang et al. [32]
proposed a variable integral PID (VIPID) controller to track the
patients’ finger trajectory which has better performance than the
conventional ones. Jones et al. [33]
used a PI controller to compensate the auxiliary torque for fingers,
and the control of either position or torque can be implemented in this
device. Polygerinos et al. [34]
presented a sliding-mode controller (SMC) for their finger
rehabilitation robot with the obvious advantage of not requiring an
explicit model of the system for the synthesis of the controller.
The
large number of degrees of freedom (DoF) and strong interjoint coupling
in human fingers make the development of finger rehabilitation robots
difficult, leading to the complex structure and difficulty in wearing
most of the existing devices. The inability of the fingers to perform
A/A motion is one of the signs of nerve damage [35],
which affects the patient’s ability to ADL. The active A/A exercise can
carry out targeted muscle strength training on the palmar interossei
and the dorsal interossei, and fully open the range of motion of the MCP
joint [36].
However, few existing robots can achieve active A/A training and cannot
fully open the joint mobility of the fingers, which affects the
rehabilitation outcome and patient experience. As for the control
strategy, it is mainly based on the PID, and the corresponding strategy
is developed for the characteristics of the developed equipment.
This paper presents the design and development of an end-effector finger rehabilitation robot (EFRR) (see Figure 1).
EFRR utilizes a fixed pulley-track module for finger F/E motion, and a
novel synchronous pulley set has been proposed for active A/A motion
driven by a single motor. This allows patients to open up their joint
mobility fully. EFRR has two thumb rehabilitation structures with
left/right symmetry, which makes it possible for functional impairment
of the left/right hand to train on the device and can reduce the cost
greatly. The design above is also the result of a comprehensive
consideration of wearing convenience, hand weight bearing, and
manufacturing cost. In terms of control strategy, an adaptive control
strategy based on fuzzy PD is designed according to the characteristics
of the EFRR, which makes it compliant during the training process and
ensures the safety of patients.
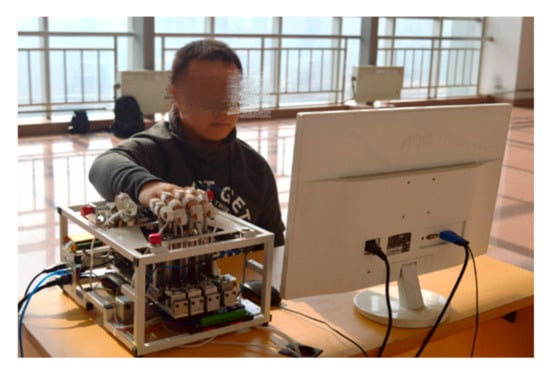
Figure 1.
Prototype of the EFRR. EFRR: end-effector finger rehabilitation robot.
The rest of this paper is organized as follows. Section 2 presents the innovative structural design of the EFRR, including the design principles and rationale; Section 3 introduces the adaptive control strategy based on fuzzy PD; Section 4 shows the preliminary experiments conducted at the EFRR and the related analysis; finally, Section 5 concludes this study and provides suggestions for future work.
More at link.
No comments:
Post a Comment