Unraveling the potential of endothelial progenitor cells as a treatment following ischemic stroke
- 1NeuroAging Laboratory (NEURAL), Clinical Neurosciences Research Laboratory (LINC), Health Research Institute of Santiago de Compostela (IDIS), Santiago de Compostela, Spain
- 2Centro Hospitalar e Universitário de Coimbra, Coimbra, Portugal
- 3Faculdade de Medicina da Universidade de Coimbra, Coimbra, Portugal
- 4Centro Neurociências e Biologia Celular, Coimbra, Portugal
- 5Neuroimaging and Biotechnology Laboratory (NOBEL), Clinical Neurosciences Research Laboratory (LINC), Health Research Institute of Santiago de Compostela (IDIS), Santiago de Compostela, Spain
- 6Neurovascular Research Laboratory, Vall d'Hebron Research Institute, Universitat Autònoma de Barcelona, Barcelona, Spain
- 7CNC-Center for Neuroscience and Cell Biology, CIBB-Centre for Innovative Biomedicine and Biotechnology, UC, Biotech Parque Tecnológico de Cantanhede, University of Coimbra, Coimbra, Portugal
Ischemic stroke is becoming one of the most common causes of death and disability in developed countries. Since current therapeutic options are quite limited, focused on acute reperfusion therapies that are hampered by a very narrow therapeutic time window, it is essential to discover novel treatments that not only stop the progression of the ischemic cascade during the acute phase,(the neuronal cascade of death I've been railing about for a decade)but also improve the recovery of stroke patients during the sub-acute or chronic phase. In this regard, several studies have shown that endothelial progenitor cells (EPCs) can repair damaged vessels as well as generate new ones following cerebrovascular damage. EPCs are circulating cells with characteristics of both endothelial cells and adult stem cells presenting the ability to differentiate into mature endothelial cells and self-renew, respectively. Moreover, EPCs have the advantage of being already present in healthy conditions as circulating cells that participate in the maintenance of the endothelium in a direct and paracrine way. In this scenario, EPCs appear as a promising target to tackle stroke by self-promoting re-endothelization, angiogenesis and vasculogenesis. Based on clinical data showing a better neurological and functional outcome in ischemic stroke patients with higher levels of circulating EPCs, novel and promising therapeutic approaches would be pharmacological treatment promoting EPCs-generation as well as EPCs-based therapies. Here, we will review the latest advances in preclinical as well as clinical research on EPCs application following stroke, not only as a single treatment but also in combination with new therapeutic approaches.
Introduction
Ischemic stroke remains as one of the leading causes of mortality and disability in Europe (1, 2). Following the ischemic insult, two large areas can be usually distinguished: the ischemic core, infarcted tissue with irreparable damage; and the penumbra area, surrounding the ischemic core, which contains hypoperfused tissue that is still viable (3). Following the occlusion of the blood vessel, the pathological paths of the ischemic cascade are activated, which eventually leads to neuronal death by either necrosis or apoptosis (4, 5). Hypoxic environment triggers glutamate excitotoxicity through a massive release of glutamate after injury, which provokes neurotoxicity by binding to N-methyl-D-aspartate receptors (NMDAR), promoting the entry of large amounts of Ca2+ in neurons (4–8). In addition, high concentrations of intracellular Ca2+ produce neuronal damage by increasing endoplasmic reticulum (ER) stress, reactive oxygen species (ROS), and depletion of ATP-depending processes (4, 5). Moreover, all together lead to the uncoupling of the mitochondrial electron chain, further increasing ROS and cellular death (4, 5). Overall, it is estimated that 2 million neurons die for every minute of obstruction (9).
Among others, mechanical thrombectomy and thrombolysis by the recombinant tissue plasminogen activator (rtPA) are effective therapeutic approaches by restoring the blood flow (10–17). However, these reperfusion therapies are hampered by a very narrow therapeutic time window, therefore it is necessary to search for alternatives and/or complementary treatments encompassed in, monitoring of cerebral homeostasis, reperfusion, neuroprotection and neurorepair strategies (12, 18, 19). In fact, angiogenesis and vasculogenesis represent two targets to obtain improvements in the outcome of patients following ischemic stroke (20–22). Whereas, angiogenesis means the sprouting of new blood vessels from uninjured ones, vasculogenesis is the de novo formation of blood vessels driven by endothelial progenitor cells (EPCs) (23). Importantly, it has been suggested that proinflammatory environments (e.g., following stroke) may enhance the proliferation and angiogenic function of EPCs (24). Therefore, both endothelial cell repair-regeneration and tissue neovascularization appear as key processes to restore the blood supply in the cortical areas affected by the vessel occlusion.
EPCs are circulating cells that exhibit both, progenitor- and stem-cell characteristics, since they have the capacity to differentiate into mature endothelial cells and self-renewing (25, 26). In the late 90's, Asahara et al. were the pioneers to identify EPCs in peripheral blood (27), and to determine their medullar origin (20). However, there is controversy nowadays regarding the origin of EPCs as both vascular and white adipocytic niches have been suggested over the years (28–31). The main function of EPCs is the maintenance of the endothelium by either releasing angiogenic growth factors or acting as a cellular reservoir for the replacement of injured endothelial cells (25, 32, 33). It is widely established that EPCs can be defined according to their angiogenic properties: early-outgrowth EPCs and late-outgrowth EPCs (25, 32, 33). On one hand, the early-outgrowth EPCs, or colony-forming unit endothelial cells (CFU-ECs), participate in the maintenance of the endothelium by the paracrine release of different pro-angiogenic factors (33). On the other hand, late-outgrowth EPCs, or endothelial colony-forming cells (ECFC), are characterized by their capacity to differentiate into mature endothelial cells, being the main player in the de novo formation of blood vessels (33). Late EPCs can also secrete several angiogenic factors, but in smaller quantities than early EPCs (34). Furthermore, these two types of EPCs can also be differentiated/classified by their in vitro characteristics: whereas early EPCs appear within a few days of culture and form colonies with spindle-shaped cells surrounding them, late EPCs appear at 2–3 weeks and show a cobblestone shape (34). Finally, kinase insert domain receptor (KDR) (also known as vascular endothelial growth factor receptor 2, VEGFR-2), CD34, and CD133 are the most common surface markers to detect EPCs (25). However, there are discrepancies regarding CD133 since it was also established as a specific marker for early EPCs (33). In addition, CD146 and von Willebrand factor (vWF) are characteristic markers of late EPCs (33). Overall, unfortunately, a unique marker to define EPCs clearly is still lacking.
Several studies have demonstrated that different factors mobilize EPCs from their niches, such as granulocyte macrophage-colony stimulating factor (GM-CSF) (35, 36), granulocyte-colony stimulating factor (G-CSF) (37, 38); vascular endothelial growth factor (VEGF) (39–42); stromal-derived factor-1α (SDF-1α) (41–44); and erythropoietin (EPO) (45). Interestingly, some of these factors (e.g., VEGF, GM-CSF, and G-CSF) are also released by EPCs as paracrine signaling, promoting angiogenesis and triggering the proliferation and migration of endothelial cells (46–48). Moreover, EPCs release different vasodilators and vasoconstrictors factors, such as nitric oxide (NO) through the endothelial nitric oxide synthase (eNOS) activity (34, 49), and the tPA (49); as well as anti-inflammatory molecules (50). In the recent years, the role of extracellular vesicles and exosomes has been described in cell-to-cell signaling (51–53). Interestingly, EPCs also release extracellular vesicles that participate in angiogenesis (53, 54). Therefore, all these features make EPCs an interesting target following cerebral ischemia as they modulate vasculogenesis and angiogenesis, either by stimulating re-endothelialization of injured vessels or by stimulating the formation of new ones (26, 55, 56). Specifically, hypoxia-inducible factor-1 (HIF-1) is up-regulated under hypoxic conditions, which activates the expression of VEGF and SDF-1α, among others; eventually inducing the homing of EPCs to the hypoxic tissues (44, 48, 55–58) (Figure 1). In addition to modulating vasculogenesis and angiogenesis, EPCs release different factors that reduce inflammation, promote neuronal survival, and maintain the myelin sheath integrity in the damaged tissue, the latter probably by regulating the survival and migration of oligodendrocytes through SDF-1α interaction (59, 60). In summary, early and late EPCs work synergistically for proper angiogenesis and revascularization: the high concentration of angiogenic factors and inflammatory cytokines in the ischemic injury area attracts early EPCs, then, early EPCs release different factors in a paracrine way that promote angiogenesis and recruit late EPCs to either restore the endothelium or form new vessels guided by the early EPCs (33) (Figure 1).
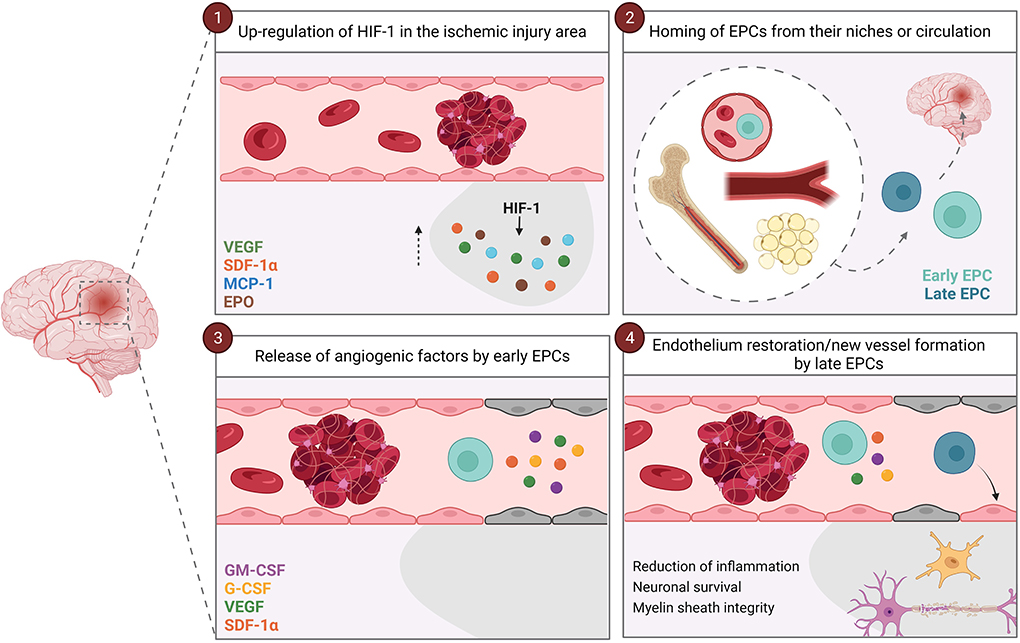
Figure 1. Vascular repair mediated by EPCs. After an ischemic stroke, HIF-1 is up-regulated and induces the expression of VEGF, SDF-1α, MCP-1 and EPO in the ischemic injury area (1). These factors induce the homing of EPCs from their niches (bone marrow, vascular niches or white adipose tissue) or circulation to the ischemic brain area (2). Firstly, early EPCs arrives at the site of injury, and paracrinally release factors that promote angiogenesis and recruit late EPCs (3). Once the late EPCs arrive at the ischemic lesion zone, they restore the endothelium or from new vessels guided by the early EPCs (4). In addition, both early and late EPCs reduce inflammation and promote neuronal survival and myelin sheath integrity. EPCs, endothelial progenitor cells; EPO, erythropoietin; G-CSF, granulocyte-colony stimulating factor; GM-CSF, granulocyte macrophage-colony stimulating factor; HIF-1, hypoxia-inducible factor-1; MCP-1, monocyte chemoattractant protein-1; SDF-1α, stromal-derived factor-1α; VEGF, vascular endothelial growth factor.
Therefore, EPCs appear as a promising target to find new therapies to overcome the ischemic damage, all thanks to their ability to both directly participate in blood vessel repair and remodeling, and indirectly release different trophic factors to stimulate the repairing processes following ischemic injury. Here, we will review the latest advances in preclinical as well as clinical research at EPCs application following stroke, not only as a single treatment but also in combination with new approaches.
No comments:
Post a Comment